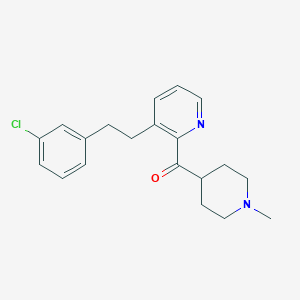
Loratadine ketone
Overview
Description
Loratadine ketone refers to key structural analogs or degradation products of loratadine, a second-generation antihistamine widely used to treat allergies. Among its related compounds, 8-Chloro-5,6-dihydro-11H-benzo[5,6]cyclohepta[1,2-b]pyridin-11-one (Loratadine Related Compound C) and tricyclic ketone (a synthesis intermediate or impurity) are prominent ketone-containing derivatives . These compounds are critical in pharmaceutical quality control, as their presence in formulations can affect drug efficacy and safety. Loratadine itself is a piperidine derivative with a carbocyclic ring system, while its ketone variants lack the piperidine substituent, altering their pharmacokinetic and pharmacodynamic properties .
Preparation Methods
Synthetic Routes and Reaction Conditions
The synthesis of Loratadine ketone typically involves multiple steps, including the formation of the piperidine ring, the introduction of the pyridine ring, and the attachment of the chlorophenyl group. Common synthetic routes may involve the use of reagents such as piperidine, pyridine, and chlorobenzene derivatives under specific reaction conditions, including controlled temperatures and the presence of catalysts.
Industrial Production Methods
Industrial production of this compound may involve large-scale chemical processes that ensure high yield and purity. Techniques such as continuous flow synthesis and the use of automated reactors can be employed to optimize the production process. The choice of solvents, catalysts, and purification methods is crucial to achieving the desired quality of the final product.
Chemical Reactions Analysis
Types of Reactions
Loratadine ketone can undergo various types of chemical reactions, including:
Oxidation: This reaction involves the addition of oxygen or the removal of hydrogen, often using oxidizing agents like potassium permanganate or hydrogen peroxide.
Reduction: This reaction involves the addition of hydrogen or the removal of oxygen, typically using reducing agents such as lithium aluminum hydride or sodium borohydride.
Substitution: This reaction involves the replacement of one functional group with another, often using reagents like halogens or alkylating agents.
Common Reagents and Conditions
The reactions of this compound often require specific reagents and conditions to proceed efficiently. For example, oxidation reactions may require acidic or basic conditions, while reduction reactions may need anhydrous solvents and inert atmospheres. Substitution reactions may be facilitated by the presence of catalysts or specific temperature and pressure conditions.
Major Products Formed
The major products formed from these reactions depend on the specific reagents and conditions used. For example, oxidation may yield ketones or carboxylic acids, reduction may produce alcohols or amines, and substitution may result in the formation of new functionalized derivatives.
Scientific Research Applications
Loratadine ketone has a wide range of scientific research applications, including:
Chemistry: It is used as a building block for the synthesis of more complex molecules and as a reagent in various organic reactions.
Biology: The compound is studied for its potential biological activities, including its interactions with enzymes and receptors.
Medicine: Research is ongoing to explore its potential therapeutic applications, such as its effects on neurological pathways and its use as a drug candidate.
Industry: The compound is used in the development of new materials and as an intermediate in the production of pharmaceuticals and agrochemicals.
Mechanism of Action
The mechanism of action of Loratadine ketone involves its interaction with specific molecular targets, such as enzymes or receptors. The compound may bind to these targets and modulate their activity, leading to various biological effects. The pathways involved in its mechanism of action can include signal transduction, gene expression, and metabolic processes.
Comparison with Similar Compounds
Structural Analogs: Loratadine vs. Hydroxyzine and Amoxapine
- Mechanistic Insight : Loratadine and hydroxyzine share antihistaminic activity but differ in sedation due to blood-brain barrier penetration . Amoxapine, though structurally distinct, shares fused aromatic features with loratadine, enabling MRSA growth inhibition in combination therapies .
Functional Analogs: Loratadine vs. Cetirizine and Dithiaden
- Key Findings :
- Loratadine suppresses oxidative burst in neutrophils less potently than dithiaden but more effectively than chlorpheniramine .
- Cetirizine has a higher sedation risk, whereas loratadine’s safety profile is superior in allergy treatment .
- Loratadine’s anticancer activity (IC50 ~15 µM in lung cancer) is linked to pyroptosis and PPARγ modulation, a unique feature among antihistamines .
Antimicrobial Adjuvants: Loratadine vs. 4-Bromocarbazole and Nafamostat
- Comparative Insight :
Pharmacokinetic Profile: Loratadine vs. Similar Poorly Soluble Drugs
- Formulation Advantage: Loratadine’s liquisolid compacts enhance dissolution at varying pH levels, unlike trichlormethiazide or tolnaftate, which rely on H-bond donors for solubility .
Biological Activity
Loratadine ketone, a derivative of the well-known antihistamine loratadine, has garnered attention for its potential biological activities beyond its primary use in treating allergic conditions. This article provides a comprehensive overview of the biological activity of this compound, including its mechanisms of action, pharmacokinetics, and comparative efficacy in various biological models.
Overview of Loratadine and Its Metabolites
Loratadine is classified as a second-generation antihistamine, primarily acting as an inverse agonist at peripheral H1 receptors. Its major active metabolite, descarboethoxyloratadine, exhibits significantly higher potency—approximately four times that of loratadine itself . The pharmacokinetics of loratadine involve extensive first-pass metabolism in the liver, primarily through cytochrome P450 enzymes (CYP3A4 and CYP2D6) which convert it into its active forms .
Table 1: Pharmacokinetic Properties of Loratadine and Its Metabolite
Parameter | Loratadine | Descarboethoxyloratadine |
---|---|---|
Half-Life | 8-14 hours | 17-24 hours |
Peak Serum Concentration | 1-2 hours post-dose | 1.5-3.5 hours post-dose |
Bioavailability | High | High |
This compound operates primarily through its interaction with H1 histamine receptors located on various cell types, including epithelial and immune cells. By binding to these receptors, it effectively reduces the symptoms associated with allergic reactions such as rhinorrhea and urticaria. Unlike first-generation antihistamines, loratadine does not readily cross the blood-brain barrier, thus minimizing central nervous system side effects like sedation .
Biological Activity Beyond Antihistamine Effects
Recent studies have indicated that loratadine and its derivatives may possess additional biological activities that could be beneficial in other therapeutic areas:
- Antiallergic Activity : this compound has been shown to inhibit bronchospasm induced by allergens in animal models. In guinea pigs, it demonstrated a significant reduction in histamine-induced bronchoconstriction compared to other antihistamines like terfenadine .
- Anticancer Potential : Research indicates that certain analogs of loratadine exhibit antiproliferative effects against various cancer cell lines. For instance, studies have shown that modifications to the loratadine structure can enhance its activity against cancer cells through mechanisms involving apoptosis and cell cycle arrest .
Case Study: Anticancer Activity
In a study examining the effects of loratadine analogs on human cancer cell lines, researchers found that specific structural modifications increased cytotoxicity against breast cancer cells by inducing apoptosis. The study highlighted the importance of molecular structure in determining biological activity and suggested further exploration into the therapeutic potential of loratadine derivatives in oncology .
Comparative Efficacy in Clinical Studies
Clinical evaluations have consistently demonstrated the efficacy of loratadine in managing allergic symptoms. A notable study assessed its effectiveness against placebo and other antihistamines over a 24-hour period. The results indicated that loratadine significantly reduced wheal and flare responses within two hours post-administration, with effects lasting up to 36 hours .
Table 2: Comparative Efficacy of Antihistamines in Clinical Trials
Antihistamine | Onset of Action | Duration of Action | Efficacy (Wheal Suppression) |
---|---|---|---|
Loratadine | 1-3 hours | Up to 36 hours | Significant |
Cetirizine | 1 hour | Up to 24 hours | Moderate |
Diphenhydramine | 15-30 minutes | 4-6 hours | High |
Q & A
Basic Research Questions
Q. What are the standard analytical methods for quantifying Loratadine ketone in pharmaceutical formulations?
- High-performance liquid chromatography (HPLC) is the most widely used method. Key parameters include:
- Mobile phase : Acetonitrile and 2% acetic acid in gradient elution to separate Loratadine from impurities (e.g., tricyclic ketone, desloratadine) .
- Validation : Accuracy (bias <2.0%), precision (%RSD <2.0%), and system suitability (retention time, peak symmetry) must be validated per ICH guidelines .
- Column selection : Cation-exchange or Agilent TC-C18 columns are effective for simultaneous quantification in complex matrices like syrups or plasma .
Q. What are the common impurities encountered in this compound synthesis, and how are they characterized?
- Major impurities include desloratadine (active metabolite), N-methyldesloratadine , and tricyclic ketone (degradation product). Characterization involves:
- Forced degradation studies : Exposure to heat, light, and acidic/alkaline conditions to simulate stability challenges .
- Spectroscopic techniques : FTIR and X-ray diffraction (XRD) confirm structural identity, while HPLC-MS identifies trace impurities .
- Linearity validation : Impurities are quantified within ranges of 0.58–58.3 µg/mL (r ≥0.9994) .
Q. How are forced degradation studies designed to assess this compound stability?
- Protocol :
Stress conditions : Acidic (HCl), alkaline (NaOH), oxidative (H₂O₂), thermal (40–80°C), and photolytic (UV light) treatments .
Sampling : Time-point assessments to track degradation kinetics.
Analytical endpoint : HPLC with gradient elution (e.g., 276 nm detection) to resolve degradation products .
- Outcome : Identification of labile functional groups (e.g., ketone moiety) and formulation strategies to enhance shelf life .
Advanced Research Questions
Q. How can response surface methodology (RSM) optimize this compound delivery systems?
- Experimental design : A 3² factorial design evaluates variables like polymer concentration (sodium alginate) and matrix-forming agents (lactose monohydrate). Key responses include bioadhesive force, disintegration time, and drug release profiles .
- Software tools : Design-Expert® generates contour plots and desirability functions to identify optimal formulations with minimal trials .
- Validation : Differential scanning calorimetry (DSC) and texture analysis (TA.XT2i) confirm compatibility and bioadhesion properties .
Q. What strategies resolve contradictions in metabolite quantification across pharmacokinetic studies?
- Method harmonization :
- Plasma preparation : Use anticoagulants (e.g., EDTA) to prevent analyte degradation .
- Chromatographic conditions : Adjust pH and column temperature to improve separation of Loratadine and desloratadine in biological matrices .
- Statistical reconciliation : Meta-analysis of dose-response relationships and sensitivity analysis to address inter-study variability .
Q. How can in vitro and in vivo models evaluate this compound’s anticancer mechanisms?
- Clinical data integration : Retrospective cohort studies (e.g., 4,522 lung cancer patients) identify associations between Loratadine use and survival outcomes .
- Cellular assays :
- Apoptosis/ferroptosis : Caspase-3 activation and lipid peroxidation assays in A549 cells .
- RNA-seq : Pathway analysis (e.g., NF-κB, MAPK) to elucidate transcriptional changes .
Q. What methodological frameworks ensure rigor in systematic reviews of this compound’s pharmacological effects?
- Research question formulation : Use PICO (Population, Intervention, Comparator, Outcome) to narrow scope (e.g., "Does Loratadine reduce histamine-induced inflammation in asthma?") .
- Bias mitigation :
- Search strategy : Multi-database queries (PubMed, Embase) with controlled vocabulary (MeSH terms) .
- Data synthesis : Random-effects models account for heterogeneity in study designs (e.g., cohort vs. case-control) .
- Reporting standards : Adhere to PRISMA guidelines for transparency in inclusion/exclusion criteria .
Properties
IUPAC Name |
[3-[2-(3-chlorophenyl)ethyl]pyridin-2-yl]-(1-methylpiperidin-4-yl)methanone | |
---|---|---|
Source | PubChem | |
URL | https://pubchem.ncbi.nlm.nih.gov | |
Description | Data deposited in or computed by PubChem | |
InChI |
InChI=1S/C20H23ClN2O/c1-23-12-9-17(10-13-23)20(24)19-16(5-3-11-22-19)8-7-15-4-2-6-18(21)14-15/h2-6,11,14,17H,7-10,12-13H2,1H3 | |
Source | PubChem | |
URL | https://pubchem.ncbi.nlm.nih.gov | |
Description | Data deposited in or computed by PubChem | |
InChI Key |
YBWTYYOASANXND-UHFFFAOYSA-N | |
Source | PubChem | |
URL | https://pubchem.ncbi.nlm.nih.gov | |
Description | Data deposited in or computed by PubChem | |
Canonical SMILES |
CN1CCC(CC1)C(=O)C2=C(C=CC=N2)CCC3=CC(=CC=C3)Cl | |
Source | PubChem | |
URL | https://pubchem.ncbi.nlm.nih.gov | |
Description | Data deposited in or computed by PubChem | |
Molecular Formula |
C20H23ClN2O | |
Source | PubChem | |
URL | https://pubchem.ncbi.nlm.nih.gov | |
Description | Data deposited in or computed by PubChem | |
Molecular Weight |
342.9 g/mol | |
Source | PubChem | |
URL | https://pubchem.ncbi.nlm.nih.gov | |
Description | Data deposited in or computed by PubChem | |
Disclaimer and Information on In-Vitro Research Products
Please be aware that all articles and product information presented on BenchChem are intended solely for informational purposes. The products available for purchase on BenchChem are specifically designed for in-vitro studies, which are conducted outside of living organisms. In-vitro studies, derived from the Latin term "in glass," involve experiments performed in controlled laboratory settings using cells or tissues. It is important to note that these products are not categorized as medicines or drugs, and they have not received approval from the FDA for the prevention, treatment, or cure of any medical condition, ailment, or disease. We must emphasize that any form of bodily introduction of these products into humans or animals is strictly prohibited by law. It is essential to adhere to these guidelines to ensure compliance with legal and ethical standards in research and experimentation.