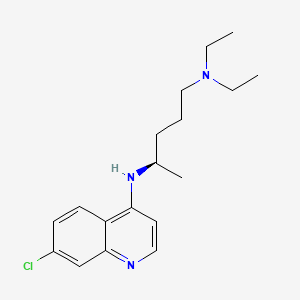
(R)-chloroquine
Overview
Description
(R)-chloroquine is a chloroquine. It is an enantiomer of a (S)-chloroquine.
Scientific Research Applications
Antiviral Research and COVID-19
- (R)-Chloroquine in Antiviral Research : Recent studies have highlighted the potential of chloroquine in treating patients infected with SARS-CoV-2, the novel coronavirus. This has brought attention to the drug's role in the field of antiviral research, considering previous experiments with chloroquine (Touret & de Lamballerie, 2020).
Biochemical Properties and Drug Repurposing
- Biochemical Properties and Therapeutic Applications : Chloroquine, known for its antimalarial effects, has also been studied for its interesting biochemical properties. This has led to its repurposing in managing various diseases, inspiring research into novel compounds based on its structure (Njaria et al., 2015).
Metabolic Pathways in Malaria Resistance
- Plasma Metabolomics in Chloroquine Resistance : A study comparing metabolic profiles of P. vivax malaria patients with chloroquine resistance identified differences in lipid, amino acids, and nucleotide metabolism pathways, suggesting potential biomarkers of resistance (Uppal et al., 2017).
Immunomodulatory and Antineoplastic Effects
- Immunomodulatory and Antineoplastic Mechanisms : Chloroquine and its analogues have shown various immunomodulatory and immunosuppressive effects, making them useful in managing rheumatic diseases, lupus, skin diseases, and different forms of cancer (Al-Bari, 2015).
Dual Role in Liver Ischemia Reperfusion Injury
- Effects on Liver Ischemia/Reperfusion Injury : Chloroquine has a dual effect in liver ischemia/reperfusion injury, providing protection against injury via inhibition of inflammatory response, but also aggravating liver injury through inhibition of autophagy (Fang et al., 2013).
Expanding Clinical Applications
- Expanded Clinical Applicability : Chloroquine's clinical applicability extends beyond malaria to include rheumatological, cardiovascular disorders, chronic kidney disease, oncology, and nonmalarial infections, due to its pleiotropic pharmacological actions (Shukla & Shukla, 2019).
Role in Anti-Cancer Chemotherapy
- Adjuvant to Anti-Cancer Chemotherapies : Chloroquine's ability to inhibit both the multidrug-resistance pump and autophagy suggests its potential as an adjuvant in anti-cancer treatments, enhancing the efficacy of chemotherapy (Pascolo, 2016).
Cardiovascular Risk Reduction
- Reduction in Cardiovascular Disease Risk : Studies indicate that chloroquine is associated with a reduced risk of cardiovascular disease in patients with rheumatic diseases (Liu et al., 2018).
Ethical Review in Off-Label Drug Research
- Ethical Review in Off-Label Use : The ethical review of off-label chloroquine research, particularly during the COVID-19 pandemic, highlights the importance of adhering to review standards and ensuring subject safety (Li et al., 2022).
Regulation of Autophagy and Sensitivity
- Autophagy Regulation and Cancer Sensitivity : Chloroquine's effect on autophagy and its sensitivity in cancer treatment is context-dependent and varies with the mutation status of oncogenic RAS in different cell types (Morgan et al., 2014).
Inhibition of T-Cell Activation
- Inhibition of CD4+ T-Cell Activation : Chloroquine suppresses the proliferation and metabolic activity of human CD4+ T-cells by modulating AP-1 signaling, suggesting its potential in treating autoimmune diseases (Schmidt et al., 2017).
Autophagy Inhibition in Endometrial Cancer
- Autophagy Inhibition in Endometrial Cancer : Chloroquine's inhibition of autophagy has been shown to suppress proliferation and overcome cisplatin resistance in endometrial cancer cells (Fukuda et al., 2015).
OATP1A2 Inhibition
- OATP1A2 Inhibition Effects : Chloroquine is a novel inhibitor of the OATP1A2 transporter, which affects substrate uptake and potentially contributes to vision impairment observed in long-term users (Xu et al., 2016).
Properties
IUPAC Name |
(4R)-4-N-(7-chloroquinolin-4-yl)-1-N,1-N-diethylpentane-1,4-diamine | |
---|---|---|
Source | PubChem | |
URL | https://pubchem.ncbi.nlm.nih.gov | |
Description | Data deposited in or computed by PubChem | |
InChI |
InChI=1S/C18H26ClN3/c1-4-22(5-2)12-6-7-14(3)21-17-10-11-20-18-13-15(19)8-9-16(17)18/h8-11,13-14H,4-7,12H2,1-3H3,(H,20,21)/t14-/m1/s1 | |
Source | PubChem | |
URL | https://pubchem.ncbi.nlm.nih.gov | |
Description | Data deposited in or computed by PubChem | |
InChI Key |
WHTVZRBIWZFKQO-CQSZACIVSA-N | |
Source | PubChem | |
URL | https://pubchem.ncbi.nlm.nih.gov | |
Description | Data deposited in or computed by PubChem | |
Canonical SMILES |
CCN(CC)CCCC(C)NC1=C2C=CC(=CC2=NC=C1)Cl | |
Source | PubChem | |
URL | https://pubchem.ncbi.nlm.nih.gov | |
Description | Data deposited in or computed by PubChem | |
Isomeric SMILES |
CCN(CC)CCC[C@@H](C)NC1=C2C=CC(=CC2=NC=C1)Cl | |
Source | PubChem | |
URL | https://pubchem.ncbi.nlm.nih.gov | |
Description | Data deposited in or computed by PubChem | |
Molecular Formula |
C18H26ClN3 | |
Source | PubChem | |
URL | https://pubchem.ncbi.nlm.nih.gov | |
Description | Data deposited in or computed by PubChem | |
DSSTOX Substance ID |
DTXSID401316905 | |
Record name | (-)-Chloroquine | |
Source | EPA DSSTox | |
URL | https://comptox.epa.gov/dashboard/DTXSID401316905 | |
Description | DSSTox provides a high quality public chemistry resource for supporting improved predictive toxicology. | |
Molecular Weight |
319.9 g/mol | |
Source | PubChem | |
URL | https://pubchem.ncbi.nlm.nih.gov | |
Description | Data deposited in or computed by PubChem | |
CAS No. |
58175-87-4 | |
Record name | (-)-Chloroquine | |
Source | CAS Common Chemistry | |
URL | https://commonchemistry.cas.org/detail?cas_rn=58175-87-4 | |
Description | CAS Common Chemistry is an open community resource for accessing chemical information. Nearly 500,000 chemical substances from CAS REGISTRY cover areas of community interest, including common and frequently regulated chemicals, and those relevant to high school and undergraduate chemistry classes. This chemical information, curated by our expert scientists, is provided in alignment with our mission as a division of the American Chemical Society. | |
Explanation | The data from CAS Common Chemistry is provided under a CC-BY-NC 4.0 license, unless otherwise stated. | |
Record name | (-)-Chloroquine | |
Source | ChemIDplus | |
URL | https://pubchem.ncbi.nlm.nih.gov/substance/?source=chemidplus&sourceid=0058175874 | |
Description | ChemIDplus is a free, web search system that provides access to the structure and nomenclature authority files used for the identification of chemical substances cited in National Library of Medicine (NLM) databases, including the TOXNET system. | |
Record name | (-)-Chloroquine | |
Source | EPA DSSTox | |
URL | https://comptox.epa.gov/dashboard/DTXSID401316905 | |
Description | DSSTox provides a high quality public chemistry resource for supporting improved predictive toxicology. | |
Record name | CHLOROQUINE, (R)- | |
Source | FDA Global Substance Registration System (GSRS) | |
URL | https://gsrs.ncats.nih.gov/ginas/app/beta/substances/WE58C2WV1T | |
Description | The FDA Global Substance Registration System (GSRS) enables the efficient and accurate exchange of information on what substances are in regulated products. Instead of relying on names, which vary across regulatory domains, countries, and regions, the GSRS knowledge base makes it possible for substances to be defined by standardized, scientific descriptions. | |
Explanation | Unless otherwise noted, the contents of the FDA website (www.fda.gov), both text and graphics, are not copyrighted. They are in the public domain and may be republished, reprinted and otherwise used freely by anyone without the need to obtain permission from FDA. Credit to the U.S. Food and Drug Administration as the source is appreciated but not required. | |
Synthesis routes and methods I
Procedure details
Synthesis routes and methods II
Procedure details
Retrosynthesis Analysis
AI-Powered Synthesis Planning: Our tool employs the Template_relevance Pistachio, Template_relevance Bkms_metabolic, Template_relevance Pistachio_ringbreaker, Template_relevance Reaxys, Template_relevance Reaxys_biocatalysis model, leveraging a vast database of chemical reactions to predict feasible synthetic routes.
One-Step Synthesis Focus: Specifically designed for one-step synthesis, it provides concise and direct routes for your target compounds, streamlining the synthesis process.
Accurate Predictions: Utilizing the extensive PISTACHIO, BKMS_METABOLIC, PISTACHIO_RINGBREAKER, REAXYS, REAXYS_BIOCATALYSIS database, our tool offers high-accuracy predictions, reflecting the latest in chemical research and data.
Strategy Settings
Precursor scoring | Relevance Heuristic |
---|---|
Min. plausibility | 0.01 |
Model | Template_relevance |
Template Set | Pistachio/Bkms_metabolic/Pistachio_ringbreaker/Reaxys/Reaxys_biocatalysis |
Top-N result to add to graph | 6 |
Feasible Synthetic Routes
Disclaimer and Information on In-Vitro Research Products
Please be aware that all articles and product information presented on BenchChem are intended solely for informational purposes. The products available for purchase on BenchChem are specifically designed for in-vitro studies, which are conducted outside of living organisms. In-vitro studies, derived from the Latin term "in glass," involve experiments performed in controlled laboratory settings using cells or tissues. It is important to note that these products are not categorized as medicines or drugs, and they have not received approval from the FDA for the prevention, treatment, or cure of any medical condition, ailment, or disease. We must emphasize that any form of bodily introduction of these products into humans or animals is strictly prohibited by law. It is essential to adhere to these guidelines to ensure compliance with legal and ethical standards in research and experimentation.