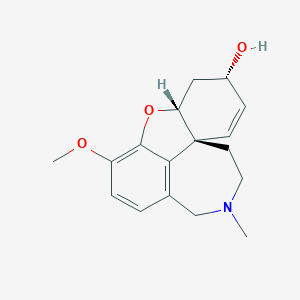
(+)-Galanthamine
Overview
Description
(+)-Galanthamine is a naturally occurring alkaloid derived from the bulbs and flowers of certain plants in the Amaryllidaceae family, such as the snowdrop (Galanthus species) and daffodil (Narcissus species). It is known for its pharmacological properties, particularly its ability to inhibit acetylcholinesterase, an enzyme that breaks down the neurotransmitter acetylcholine. This makes this compound a valuable compound in the treatment of neurological disorders, such as Alzheimer’s disease.
Scientific Research Applications
(+)-Galanthamine has a wide range of scientific research applications, including:
Chemistry: It is used as a chiral building block in the synthesis of other complex molecules.
Biology: It serves as a tool to study the role of acetylcholinesterase in neurotransmission.
Medicine: this compound is used in the treatment of Alzheimer’s disease due to its acetylcholinesterase inhibitory activity. It helps to increase the levels of acetylcholine in the brain, thereby improving cognitive function in patients.
Industry: It is used in the pharmaceutical industry for the development of drugs targeting neurological disorders.
Mechanism of Action
Target of Action
The primary target of (+)-Galanthamine is the acetylcholinesterase enzyme, which plays a crucial role in the nervous system. This enzyme is responsible for the breakdown of acetylcholine, a neurotransmitter that is involved in memory and learning processes. By targeting acetylcholinesterase, this compound helps to increase the levels of acetylcholine in the brain, thereby enhancing cognitive function .
Mode of Action
This compound interacts with its target, the acetylcholinesterase enzyme, by binding to it and inhibiting its activity. This inhibition prevents the breakdown of acetylcholine, leading to an increase in its concentration in the synaptic cleft. The increased levels of acetylcholine enhance the transmission of signals in the neurons, which is beneficial for memory and cognition .
Biochemical Pathways
The action of this compound affects the cholinergic pathway in the brain. This pathway involves the synthesis, release, and breakdown of acetylcholine. By inhibiting acetylcholinesterase, this compound disrupts the normal flow of this pathway, leading to an accumulation of acetylcholine. This results in enhanced cholinergic transmission, which has downstream effects on cognitive function .
Pharmacokinetics
The pharmacokinetics of this compound involve its absorption, distribution, metabolism, and excretion (ADME). After oral administration, this compound is well absorbed in the gastrointestinal tract. It is widely distributed throughout the body, including the brain, which is its site of action. This compound is metabolized in the liver and excreted in the urine. The pharmacokinetic properties of this compound contribute to its bioavailability and therapeutic effects .
Result of Action
The molecular and cellular effects of this compound’s action include increased levels of acetylcholine in the brain and enhanced cholinergic transmission. These effects lead to improvements in cognitive function, particularly in individuals with neurodegenerative disorders such as Alzheimer’s disease. The action of this compound can also result in side effects, which can include nausea, vomiting, and diarrhea .
Action Environment
Environmental factors can influence the action, efficacy, and stability of this compound. For example, factors such as temperature and pH can affect the stability of the compound. Additionally, individual factors such as age, genetic makeup, and health status can influence how this compound is metabolized and excreted, thereby affecting its efficacy .
Preparation Methods
Synthetic Routes and Reaction Conditions
The synthesis of (+)-Galanthamine involves several steps, starting from simpler organic molecules. One common synthetic route begins with the compound norbelladine, which undergoes a series of reactions including oxidation, cyclization, and reduction to form the galanthamine structure. The key steps in this synthesis include:
Oxidation: Norbelladine is oxidized to form an imine intermediate.
Cyclization: The imine undergoes cyclization to form a tetrahydroisoquinoline structure.
Reduction: The tetrahydroisoquinoline is reduced to form the final galanthamine structure.
Industrial Production Methods
Industrial production of this compound typically involves extraction from natural sources, such as the bulbs of Galanthus species. The extraction process includes:
Harvesting: Bulbs are harvested and cleaned.
Extraction: The alkaloids are extracted using solvents such as ethanol or methanol.
Purification: The crude extract is purified using techniques like chromatography to isolate this compound.
Chemical Reactions Analysis
(+)-Galanthamine undergoes various chemical reactions, including:
Oxidation: It can be oxidized to form galanthamine N-oxide.
Reduction: Reduction of this compound can yield dihydrogalanthamine.
Substitution: It can undergo substitution reactions, particularly at the nitrogen atom, to form various derivatives.
Common reagents and conditions used in these reactions include oxidizing agents like hydrogen peroxide for oxidation, reducing agents like sodium borohydride for reduction, and alkylating agents for substitution reactions. The major products formed from these reactions include galanthamine N-oxide, dihydrogalanthamine, and various substituted derivatives.
Comparison with Similar Compounds
(+)-Galanthamine is often compared with other acetylcholinesterase inhibitors, such as donepezil, rivastigmine, and physostigmine. While all these compounds share the common mechanism of inhibiting acetylcholinesterase, this compound is unique due to its dual action on both acetylcholinesterase and nicotinic acetylcholine receptors. This dual action may provide additional therapeutic benefits in the treatment of neurological disorders.
Similar Compounds
Donepezil: Another acetylcholinesterase inhibitor used in the treatment of Alzheimer’s disease.
Rivastigmine: A reversible acetylcholinesterase inhibitor also used for Alzheimer’s disease.
Physostigmine: A reversible inhibitor of acetylcholinesterase, used in the treatment of glaucoma and myasthenia gravis.
Properties
IUPAC Name |
(1R,12R,14S)-9-methoxy-4-methyl-11-oxa-4-azatetracyclo[8.6.1.01,12.06,17]heptadeca-6(17),7,9,15-tetraen-14-ol | |
---|---|---|
Source | PubChem | |
URL | https://pubchem.ncbi.nlm.nih.gov | |
Description | Data deposited in or computed by PubChem | |
InChI |
InChI=1S/C17H21NO3/c1-18-8-7-17-6-5-12(19)9-14(17)21-16-13(20-2)4-3-11(10-18)15(16)17/h3-6,12,14,19H,7-10H2,1-2H3/t12-,14-,17-/m1/s1 | |
Source | PubChem | |
URL | https://pubchem.ncbi.nlm.nih.gov | |
Description | Data deposited in or computed by PubChem | |
InChI Key |
ASUTZQLVASHGKV-SUYBPPKGSA-N | |
Source | PubChem | |
URL | https://pubchem.ncbi.nlm.nih.gov | |
Description | Data deposited in or computed by PubChem | |
Canonical SMILES |
CN1CCC23C=CC(CC2OC4=C(C=CC(=C34)C1)OC)O | |
Source | PubChem | |
URL | https://pubchem.ncbi.nlm.nih.gov | |
Description | Data deposited in or computed by PubChem | |
Isomeric SMILES |
CN1CC[C@]23C=C[C@H](C[C@H]2OC4=C(C=CC(=C34)C1)OC)O | |
Source | PubChem | |
URL | https://pubchem.ncbi.nlm.nih.gov | |
Description | Data deposited in or computed by PubChem | |
Molecular Formula |
C17H21NO3 | |
Source | PubChem | |
URL | https://pubchem.ncbi.nlm.nih.gov | |
Description | Data deposited in or computed by PubChem | |
Molecular Weight |
287.35 g/mol | |
Source | PubChem | |
URL | https://pubchem.ncbi.nlm.nih.gov | |
Description | Data deposited in or computed by PubChem | |
CAS No. |
60384-53-4 | |
Record name | Galanthamine, (+)- | |
Source | ChemIDplus | |
URL | https://pubchem.ncbi.nlm.nih.gov/substance/?source=chemidplus&sourceid=0060384534 | |
Description | ChemIDplus is a free, web search system that provides access to the structure and nomenclature authority files used for the identification of chemical substances cited in National Library of Medicine (NLM) databases, including the TOXNET system. | |
Record name | GALANTAMINE, (+)- | |
Source | FDA Global Substance Registration System (GSRS) | |
URL | https://gsrs.ncats.nih.gov/ginas/app/beta/substances/8L3T05AQ82 | |
Description | The FDA Global Substance Registration System (GSRS) enables the efficient and accurate exchange of information on what substances are in regulated products. Instead of relying on names, which vary across regulatory domains, countries, and regions, the GSRS knowledge base makes it possible for substances to be defined by standardized, scientific descriptions. | |
Explanation | Unless otherwise noted, the contents of the FDA website (www.fda.gov), both text and graphics, are not copyrighted. They are in the public domain and may be republished, reprinted and otherwise used freely by anyone without the need to obtain permission from FDA. Credit to the U.S. Food and Drug Administration as the source is appreciated but not required. | |
Synthesis routes and methods I
Procedure details
Synthesis routes and methods II
Procedure details
Retrosynthesis Analysis
AI-Powered Synthesis Planning: Our tool employs the Template_relevance Pistachio, Template_relevance Bkms_metabolic, Template_relevance Pistachio_ringbreaker, Template_relevance Reaxys, Template_relevance Reaxys_biocatalysis model, leveraging a vast database of chemical reactions to predict feasible synthetic routes.
One-Step Synthesis Focus: Specifically designed for one-step synthesis, it provides concise and direct routes for your target compounds, streamlining the synthesis process.
Accurate Predictions: Utilizing the extensive PISTACHIO, BKMS_METABOLIC, PISTACHIO_RINGBREAKER, REAXYS, REAXYS_BIOCATALYSIS database, our tool offers high-accuracy predictions, reflecting the latest in chemical research and data.
Strategy Settings
Precursor scoring | Relevance Heuristic |
---|---|
Min. plausibility | 0.01 |
Model | Template_relevance |
Template Set | Pistachio/Bkms_metabolic/Pistachio_ringbreaker/Reaxys/Reaxys_biocatalysis |
Top-N result to add to graph | 6 |
Feasible Synthetic Routes
Disclaimer and Information on In-Vitro Research Products
Please be aware that all articles and product information presented on BenchChem are intended solely for informational purposes. The products available for purchase on BenchChem are specifically designed for in-vitro studies, which are conducted outside of living organisms. In-vitro studies, derived from the Latin term "in glass," involve experiments performed in controlled laboratory settings using cells or tissues. It is important to note that these products are not categorized as medicines or drugs, and they have not received approval from the FDA for the prevention, treatment, or cure of any medical condition, ailment, or disease. We must emphasize that any form of bodily introduction of these products into humans or animals is strictly prohibited by law. It is essential to adhere to these guidelines to ensure compliance with legal and ethical standards in research and experimentation.