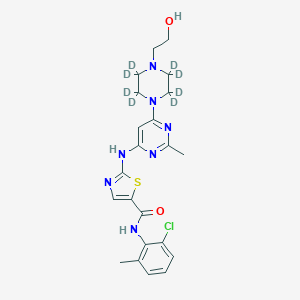
Dasatinib-d8
Overview
Description
Dasatinib-d8 is a deuterated form of dasatinib, a potent tyrosine kinase inhibitor used primarily in the treatment of chronic myeloid leukemia and Philadelphia chromosome-positive acute lymphoblastic leukemia . The deuterated version, this compound, is often used as an internal standard in mass spectrometry due to its similar chemical properties to dasatinib but with a distinct mass difference .
Scientific Research Applications
Dasatinib-d8 is widely used in scientific research, particularly in the fields of chemistry, biology, and medicine . In chemistry, it serves as an internal standard in mass spectrometry, allowing for accurate quantification of dasatinib in various samples . In biology and medicine, this compound is used to study the pharmacokinetics and metabolism of dasatinib, providing insights into its absorption, distribution, metabolism, and excretion . Additionally, it is used in drug development and clinical research to evaluate the efficacy and safety of dasatinib and related compounds .
Mechanism of Action
Target of Action
Dasatinib is a potent, oral multi-targeted tyrosine kinase inhibitor . Its primary targets include BCR-ABL, SRC-family kinases (SRC, LCK, YES, FYN), c-KIT, EPHA2, and PDGFRβ . These targets play crucial roles in the pathogenesis of various cancers, including chronic myelogenous leukemia (CML) and acute lymphoblastic leukemia (ALL) .
Mode of Action
Dasatinib interacts with its targets by inhibiting their tyrosine kinase activity . It is particularly effective against the BCR-ABL tyrosine kinase, which is associated with uncontrolled activity in CML and ALL cases . Unlike imatinib, another tyrosine kinase inhibitor, dasatinib inhibits both the active and inactive conformations of the ABL kinase domain . This makes dasatinib a valuable therapeutic alternative for patients with cancers that have developed imatinib-resistance .
Biochemical Pathways
Dasatinib affects several biochemical pathways. It inhibits the BCR-ABL and SRC-family kinases, leading to the downregulation of several signaling pathways such as HCG/FAK/SRC and Ras/Raf/ERK/P13K/Akt . These pathways are involved in cell proliferation, survival, and differentiation, and their inhibition can lead to the suppression of cancer cell growth .
Pharmacokinetics
Dasatinib is rapidly absorbed, with the time for maximal serum concentration varying between 0.25 and 1.5 hours . It is eliminated through cytochrome P450 (CYP) 3A4-mediated metabolism, with a terminal half-life of 3–4 hours . Only 20% of the oral dose is recovered unchanged in feces and urine . It is also subject to drug interactions with CYP3A4 inducers or inhibitors .
Result of Action
Dasatinib’s action results in the inhibition of cancer cell growth and induction of apoptosis . It suppresses the expression of markers related to cell cycle and increases the levels of markers involved in cell apoptosis . In patient-derived xenografts, dasatinib significantly inhibited the growth of tumors .
Action Environment
Environmental factors such as age and gastric pH can influence the action of dasatinib . For instance, dasatinib’s clearance is associated with age, suggesting that dosage can be appropriately reduced according to the increase of age . Additionally, dasatinib’s solubility decreases at pH > 4, making it susceptible to alteration by pH-modifying agents .
Safety and Hazards
If inhaled, move the victim into fresh air. If breathing is difficult, give oxygen. If not breathing, give artificial respiration and consult a doctor immediately . Following skin contact, take off contaminated clothing immediately. Wash off with soap and plenty of water. Consult a doctor . Following eye contact, rinse with pure water for at least 15 minutes. Consult a doctor . Following ingestion, rinse mouth with water. Do not induce vomiting. Never give anything by mouth to an unconscious person. Call a doctor or Poison Control Center immediately .
Future Directions
Dasatinib-d8, as a potent inhibitor of non-receptor tyrosine kinases, has potential therapeutic value in diseases characterized by elevated levels of these kinases, including some forms of cancer and fibrotic disease . Therapeutic drug monitoring (TDM) is gradually becoming a practical tool to achieve the goal of individualized medicine for patients receiving targeted drugs . With the help of TDM, these patients who maintain response while have minimum adverse events may achieve long-term survival .
Biochemical Analysis
Biochemical Properties
Dasatinib-d8 is known to inhibit a broad array of kinases, including BCR-ABL, SRC, c-KIT, PDGFR-α, PDGFR-β, and ephrin receptor kinases . The main targets of this compound are BCR/Abl (the “Philadelphia chromosome”), Src, c-Kit, ephrin receptors, and several other tyrosine kinases . This wide range of interactions allows this compound to play a significant role in various biochemical reactions.
Cellular Effects
This compound has shown to have significant effects on various types of cells and cellular processes . It influences cell function by impacting cell signaling pathways, gene expression, and cellular metabolism . For instance, this compound has been shown to influence NK cell cytotoxicity .
Molecular Mechanism
This compound exerts its effects at the molecular level through various mechanisms. It is an ATP-competitive protein tyrosine kinase inhibitor . Its main mechanism of action involves binding interactions with biomolecules, enzyme inhibition or activation, and changes in gene expression .
Temporal Effects in Laboratory Settings
In laboratory settings, the effects of this compound have been observed to change over time . It has been noted for its stability and any long-term effects on cellular function observed in in vitro or in vivo studies .
Dosage Effects in Animal Models
The effects of this compound have been studied in animal models, and it has been observed that these effects vary with different dosages . These studies have provided valuable information on any threshold effects, as well as any toxic or adverse effects at high doses .
Metabolic Pathways
This compound is involved in various metabolic pathways. It is eliminated through cytochrome P450 (CYP) 3A4-mediated metabolism . This interaction with enzymes and cofactors plays a significant role in its metabolic activity.
Transport and Distribution
This compound is well absorbed orally and extensively distributed to the extravascular tissues . It interacts with transporters or binding proteins, which affects its localization or accumulation within cells and tissues .
Preparation Methods
Synthetic Routes and Reaction Conditions
The synthesis of Dasatinib-d8 involves the incorporation of deuterium atoms into the dasatinib molecule. This is typically achieved through a series of chemical reactions that replace hydrogen atoms with deuterium. One common method involves the use of deuterated reagents in the synthesis process . For example, the use of deuterated solvents and deuterated reducing agents can facilitate the incorporation of deuterium into the final product.
Industrial Production Methods
Industrial production of this compound follows similar principles but on a larger scale. The process involves the use of high-purity deuterated reagents and solvents to ensure the consistent incorporation of deuterium atoms. The final product is then purified using techniques such as liquid chromatography to achieve the desired purity and isotopic enrichment .
Chemical Reactions Analysis
Types of Reactions
Dasatinib-d8, like its non-deuterated counterpart, undergoes various chemical reactions, including oxidation, reduction, and substitution reactions . These reactions are essential for its metabolism and function within biological systems.
Common Reagents and Conditions
Common reagents used in the reactions involving this compound include oxidizing agents like hydrogen peroxide, reducing agents such as sodium borohydride, and various nucleophiles for substitution reactions . The conditions for these reactions typically involve controlled temperatures and pH levels to ensure the desired reaction pathways.
Major Products Formed
The major products formed from the reactions of this compound depend on the specific reaction conditions. For example, oxidation reactions may produce hydroxylated metabolites, while reduction reactions can lead to the formation of deuterated analogs of dasatinib .
Comparison with Similar Compounds
Dasatinib-d8 is unique due to its deuterium incorporation, which distinguishes it from other similar compounds such as imatinib and nilotinib . These compounds also inhibit tyrosine kinases but differ in their chemical structures and specific kinase targets . For example, imatinib primarily targets BCR-ABL, while nilotinib has a broader spectrum of kinase inhibition . The deuterium incorporation in this compound provides advantages in mass spectrometry analysis, making it a preferred internal standard for studying dasatinib and its analogs .
Similar Compounds
Imatinib: A tyrosine kinase inhibitor primarily targeting BCR-ABL.
Nilotinib: A tyrosine kinase inhibitor with a broader spectrum of kinase inhibition.
Bosutinib: Another tyrosine kinase inhibitor used in the treatment of chronic myeloid leukemia.
Ponatinib: A multi-kinase inhibitor with activity against BCR-ABL and other kinases.
Properties
IUPAC Name |
N-(2-chloro-6-methylphenyl)-2-[[2-methyl-6-[2,2,3,3,5,5,6,6-octadeuterio-4-(2-hydroxyethyl)piperazin-1-yl]pyrimidin-4-yl]amino]-1,3-thiazole-5-carboxamide | |
---|---|---|
Source | PubChem | |
URL | https://pubchem.ncbi.nlm.nih.gov | |
Description | Data deposited in or computed by PubChem | |
InChI |
InChI=1S/C22H26ClN7O2S/c1-14-4-3-5-16(23)20(14)28-21(32)17-13-24-22(33-17)27-18-12-19(26-15(2)25-18)30-8-6-29(7-9-30)10-11-31/h3-5,12-13,31H,6-11H2,1-2H3,(H,28,32)(H,24,25,26,27)/i6D2,7D2,8D2,9D2 | |
Source | PubChem | |
URL | https://pubchem.ncbi.nlm.nih.gov | |
Description | Data deposited in or computed by PubChem | |
InChI Key |
ZBNZXTGUTAYRHI-COMRDEPKSA-N | |
Source | PubChem | |
URL | https://pubchem.ncbi.nlm.nih.gov | |
Description | Data deposited in or computed by PubChem | |
Canonical SMILES |
CC1=C(C(=CC=C1)Cl)NC(=O)C2=CN=C(S2)NC3=CC(=NC(=N3)C)N4CCN(CC4)CCO | |
Source | PubChem | |
URL | https://pubchem.ncbi.nlm.nih.gov | |
Description | Data deposited in or computed by PubChem | |
Isomeric SMILES |
[2H]C1(C(N(C(C(N1CCO)([2H])[2H])([2H])[2H])C2=NC(=NC(=C2)NC3=NC=C(S3)C(=O)NC4=C(C=CC=C4Cl)C)C)([2H])[2H])[2H] | |
Source | PubChem | |
URL | https://pubchem.ncbi.nlm.nih.gov | |
Description | Data deposited in or computed by PubChem | |
Molecular Formula |
C22H26ClN7O2S | |
Source | PubChem | |
URL | https://pubchem.ncbi.nlm.nih.gov | |
Description | Data deposited in or computed by PubChem | |
DSSTOX Substance ID |
DTXSID30649385 | |
Record name | N-(2-Chloro-6-methylphenyl)-2-({6-[4-(2-hydroxyethyl)(~2~H_8_)piperazin-1-yl]-2-methylpyrimidin-4-yl}amino)-1,3-thiazole-5-carboxamide | |
Source | EPA DSSTox | |
URL | https://comptox.epa.gov/dashboard/DTXSID30649385 | |
Description | DSSTox provides a high quality public chemistry resource for supporting improved predictive toxicology. | |
Molecular Weight |
496.1 g/mol | |
Source | PubChem | |
URL | https://pubchem.ncbi.nlm.nih.gov | |
Description | Data deposited in or computed by PubChem | |
CAS No. |
1132093-70-9 | |
Record name | N-(2-Chloro-6-methylphenyl)-2-({6-[4-(2-hydroxyethyl)(~2~H_8_)piperazin-1-yl]-2-methylpyrimidin-4-yl}amino)-1,3-thiazole-5-carboxamide | |
Source | EPA DSSTox | |
URL | https://comptox.epa.gov/dashboard/DTXSID30649385 | |
Description | DSSTox provides a high quality public chemistry resource for supporting improved predictive toxicology. | |
Record name | N-(2-chloro-6-methyl-phenyl)-2-[[2-methyl-6-[2,2,3,3,5,5,6,6-octadeuterio-4-(2-hydroxyethyl)piperazin-1-yl]pyrimidin-4-yl]methyl]thiazole-5-carboxamide | |
Source | European Chemicals Agency (ECHA) | |
URL | https://echa.europa.eu/information-on-chemicals | |
Description | The European Chemicals Agency (ECHA) is an agency of the European Union which is the driving force among regulatory authorities in implementing the EU's groundbreaking chemicals legislation for the benefit of human health and the environment as well as for innovation and competitiveness. | |
Explanation | Use of the information, documents and data from the ECHA website is subject to the terms and conditions of this Legal Notice, and subject to other binding limitations provided for under applicable law, the information, documents and data made available on the ECHA website may be reproduced, distributed and/or used, totally or in part, for non-commercial purposes provided that ECHA is acknowledged as the source: "Source: European Chemicals Agency, http://echa.europa.eu/". Such acknowledgement must be included in each copy of the material. ECHA permits and encourages organisations and individuals to create links to the ECHA website under the following cumulative conditions: Links can only be made to webpages that provide a link to the Legal Notice page. | |
Synthesis routes and methods I
Procedure details
Synthesis routes and methods II
Procedure details
Synthesis routes and methods III
Procedure details
Synthesis routes and methods IV
Procedure details
Retrosynthesis Analysis
AI-Powered Synthesis Planning: Our tool employs the Template_relevance Pistachio, Template_relevance Bkms_metabolic, Template_relevance Pistachio_ringbreaker, Template_relevance Reaxys, Template_relevance Reaxys_biocatalysis model, leveraging a vast database of chemical reactions to predict feasible synthetic routes.
One-Step Synthesis Focus: Specifically designed for one-step synthesis, it provides concise and direct routes for your target compounds, streamlining the synthesis process.
Accurate Predictions: Utilizing the extensive PISTACHIO, BKMS_METABOLIC, PISTACHIO_RINGBREAKER, REAXYS, REAXYS_BIOCATALYSIS database, our tool offers high-accuracy predictions, reflecting the latest in chemical research and data.
Strategy Settings
Precursor scoring | Relevance Heuristic |
---|---|
Min. plausibility | 0.01 |
Model | Template_relevance |
Template Set | Pistachio/Bkms_metabolic/Pistachio_ringbreaker/Reaxys/Reaxys_biocatalysis |
Top-N result to add to graph | 6 |
Feasible Synthetic Routes
Q1: How does the use of Dasatinib-d8 contribute to the reliability of Dasatinib quantification in pharmacokinetic studies?
A2: The incorporation of this compound as an internal standard in LC-MS/MS analysis significantly enhances the accuracy and reliability of Dasatinib quantification, particularly in complex matrices like plasma. [] This is because it corrects for potential variations during sample processing and ionization efficiency, which can impact the signal intensity of the analyte. By comparing the signal of Dasatinib to the known concentration of this compound, researchers can obtain more precise and reproducible measurements of Dasatinib concentrations in biological samples. This enhanced accuracy is essential for understanding the absorption, distribution, metabolism, and excretion profile of Dasatinib in preclinical and clinical settings.
Disclaimer and Information on In-Vitro Research Products
Please be aware that all articles and product information presented on BenchChem are intended solely for informational purposes. The products available for purchase on BenchChem are specifically designed for in-vitro studies, which are conducted outside of living organisms. In-vitro studies, derived from the Latin term "in glass," involve experiments performed in controlled laboratory settings using cells or tissues. It is important to note that these products are not categorized as medicines or drugs, and they have not received approval from the FDA for the prevention, treatment, or cure of any medical condition, ailment, or disease. We must emphasize that any form of bodily introduction of these products into humans or animals is strictly prohibited by law. It is essential to adhere to these guidelines to ensure compliance with legal and ethical standards in research and experimentation.