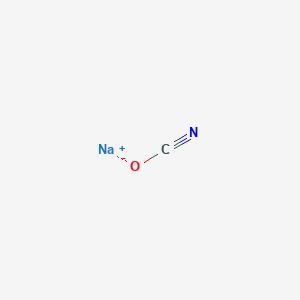
Sodium cyanate
- Click on QUICK INQUIRY to receive a quote from our team of experts.
- With the quality product at a COMPETITIVE price, you can focus more on your research.
Overview
Description
Sodium cyanate (NaOCN) is an inorganic compound with the chemical formula NaOCN. Initially investigated as a therapeutic agent for sickle-cell disease due to its ability to carbamylate hemoglobin and increase oxygen affinity, it transitioned to a research tool in physiology, particularly for studying oxygen transport under normoxic and hypoxic conditions . Its primary mechanism involves carbamylation of the NH2-terminal valine of hemoglobin, which shifts the oxygen dissociation curve to the left without significant toxicity in chronic administration . Industrially, NaOCN is used in agrochemicals, metallurgy, pharmaceuticals, and textile industries .
Preparation Methods
Synthetic Routes and Reaction Conditions: Sodium cyanate can be synthesized through the reaction of urea with sodium carbonate at elevated temperatures. The reaction proceeds as follows: [ 2 \text{OC(NH}_2\text{)}_2 + \text{Na}_2\text{CO}_3 \rightarrow 2 \text{Na(NCO)} + \text{CO}_2 + 2 \text{NH}_3 + \text{H}_2\text{O} ] An intermediate, sodium allophanate, is observed during this process: [ \text{H}_2\text{NC(O)NHCO}_2\text{Na} \rightarrow \text{NaOCN} + \text{NH}_3 + \text{CO}_2 ]
Industrial Production Methods: Industrially, this compound is produced by heating a mixture of urea and sodium carbonate. This method is preferred due to its efficiency and the availability of raw materials .
Chemical Reactions Analysis
Types of Reactions: Sodium cyanate undergoes various chemical reactions, including:
Oxidation: this compound can be oxidized to form cyanic acid.
Substitution: It reacts with hydrochloric acid to produce cyanic acid and sodium chloride: [ \text{NaOCN} + \text{HCl} \rightarrow \text{HOCN} + \text{NaCl} ]
Condensation: Cyanic acid formed in situ can condense with amines to produce unsymmetrical ureas: [ \text{HOCN} + \text{RNH}_2 \rightarrow \text{RNHC(O)NH}_2 ]
Common Reagents and Conditions:
Oxidizing Agents: Mild oxidizing agents such as lead oxide can be used to prepare this compound from cyanides.
Acids: Hydrochloric acid is commonly used in substitution reactions.
Major Products:
Cyanic Acid: Formed during substitution reactions.
Unsymmetrical Ureas: Produced through condensation reactions with amines.
Scientific Research Applications
Agricultural Applications
Herbicide Use
Sodium cyanate is recognized for its herbicidal properties. It acts rapidly against annual weeds, demonstrating effectiveness within three to four days post-application. The compound breaks down into carbonate and ammonia, enhancing soil fertility and providing a nitrogen source for crops . Its rapid degradation makes it an environmentally friendly option compared to persistent herbicides.
Table 1: Herbicidal Efficacy of this compound
Weed Type | Time to Death (Days) | Mechanism of Action |
---|---|---|
Annual Grasses | 3-4 | Rapid cell death through protein denaturation |
Broadleaf Weeds | 3-4 | Disruption of metabolic processes |
Biomedical Research
Therapeutic Potential
Initially explored as a therapeutic agent for sickle cell disease, this compound was found to induce a leftward shift in the oxygen dissociation curve. This property has made it valuable in physiological research, particularly in studies related to oxygen transport under varying oxygen conditions (normoxic and hypoxic) . Although it did not become a standard treatment, its role as a pharmacological tool remains significant.
Case Study: Sickle Cell Disease Research
In studies involving animal models, this compound was administered to observe its effects on hemoglobin's affinity for oxygen. Results indicated that this compound could potentially improve oxygen delivery in hypoxic environments, supporting further exploration in high-altitude physiology .
Chemical Synthesis
Raw Material for Organic Synthesis
this compound serves as an important raw material in organic synthesis. It can be utilized to produce various chemical compounds through reactions such as the formation of isocyanates, which are precursors for polyurethanes and other polymers .
Table 2: Chemical Reactions Involving this compound
Reaction Type | Product | Notes |
---|---|---|
Hydrolysis | Isocyanic acid | Key intermediate in polymer production |
Reaction with amines | Ureas | Important for pharmaceutical synthesis |
Environmental Impact and Safety
This compound has been evaluated for its environmental impact, particularly regarding its degradation products. Studies indicate that while it poses risks at high doses—potentially damaging organs such as the liver and spleen—it is generally safe when used according to recommended guidelines .
Industrial Applications
Case Hardening Agent
In metallurgy, this compound is used as a case hardening agent for steel. This application enhances the surface hardness of steel components, making them more resistant to wear and corrosion .
Mechanism of Action
Sodium cyanate exerts its effects through the carbamylation of proteins. This process involves the addition of the cyanate group to the terminal valine residues of hemoglobin, which alters the protein’s structure and function. This mechanism is particularly relevant in the context of sickle cell disease, where carbamylation prevents the sickling of red blood cells .
Comparison with Similar Compounds
Comparative Analysis with Similar Compounds
Chemical and Structural Comparisons
Cyanide (CN⁻)
- Toxicity : Sodium cyanate (LD₅₀: 260 mg/kg in mice) is significantly less toxic than sodium cyanide (LD₅₀: ~6 mg/kg in rats). Cyanide inhibits cytochrome oxidase, causing rapid systemic toxicity, whereas cyanate induces drowsiness, diuresis, and reversible carbamylation without cyanide conversion .
- Cyanate’s terminal convulsions and diuresis contrast with cyanide’s rapid lethality and metabolic inhibition .
Urea (NH₂CONH₂)
- Hydrolysis : Cyanate hydrolyzes to urea in aqueous solutions but exhibits distinct pharmacological effects. Unlike urea, cyanate induces prolonged drowsiness and sleep in rats, suggesting unique interactions with the central nervous system .
Thiocyanate (SCN⁻)
- Reactivity: Thiocyanate shares structural similarity but differs in sulfur substitution.
Isocyanates (e.g., tert-butyl isocyanate, benzyl isothiocyanate)
- Chemoprevention: Like cyanate, these compounds inhibit carcinogen-induced neoplasia post-exposure. However, cyanate’s selectivity for tumor protein synthesis (via liver-activated metabolites) distinguishes it from other isocyanates .
Carbamylation Specificity
- Reversible Sulfhydryl Blocking : Unlike irreversible sulfhydryl blockers, cyanate forms reversible S-carbamylcysteine, stable below pH 5 but labile at pH 8, enabling controlled protein modification .
Agrochemicals vs. Metallurgy
- Cyanate : Used in herbicides and nitrogen stabilization.
- Cyanide : Employed in gold extraction (cyanidation), highlighting divergent industrial roles despite structural similarity .
Environmental Cycling
- Soil Cyanate Cycling : Cyanate gross production rates in soils (26.0 pmol g⁻¹ d.w. d⁻¹) are orders of magnitude lower than nitrification rates. Its cycling parallels urea degradation but occurs at lower concentrations .
Data Tables
Table 1: Toxicity and Pharmacokinetic Comparison
Biological Activity
Sodium cyanate (NaOCN) is a compound that has garnered attention for its diverse biological activities, particularly in the context of medical research. Initially explored as a potential therapeutic agent for sickle cell disease, this compound has demonstrated various effects on oxygen transport, erythrocyte survival, and even potential anticancer properties. This article delves into the biological activity of this compound, highlighting key research findings, case studies, and relevant data.
Overview of this compound
This compound is an inorganic compound that can be synthesized from the reaction of sodium hydroxide with cyanamide. It is known for its ability to carbamylate proteins, which modifies their structure and function. This property has made it a valuable tool in physiological research, particularly in studies related to hemoglobin and oxygen transport.
- Oxygen Transport and Hemoglobin Affinity : Research indicates that this compound can induce a leftward shift in the oxygen dissociation curve of hemoglobin. This shift enhances hemoglobin's affinity for oxygen, which is particularly beneficial in hypoxic conditions. Studies have shown that animals treated with this compound exhibit increased survival rates under hypoxic stress due to improved oxygen transport capabilities .
- Erythrocyte Survival : this compound has been shown to improve the survival of sickle cell erythrocytes in vitro and in vivo. In clinical studies, patients with sickle cell disease demonstrated increased erythrocyte survival when treated with this compound, although it did not completely prevent sickle cell crises .
- Antimicrobial Activity : At specific concentrations, this compound has been observed to inhibit the bactericidal activity of neutrophils against pathogens such as Staphylococcus epidermidis and Escherichia coli. This suggests a potential role in modulating immune responses .
- Anticancer Properties : this compound has also been investigated for its effects on tumor tissues. A Phase I clinical trial indicated that it selectively suppresses amino acid incorporation for protein synthesis in tumor cells, highlighting its potential as an anticancer agent .
Case Studies
- High Altitude Physiology : A study involving mice showed that those treated with this compound had significantly higher hemoglobin concentrations and better survival rates when exposed to high-altitude hypoxia compared to untreated controls. The treated mice maintained a slower heart rate and exhibited less weight loss under stress conditions .
- Sickle Cell Disease Management : Clinical trials have demonstrated that this compound can improve the bioavailability of treatments for sickle cell disease when administered with antacids, enhancing patient outcomes by improving erythrocyte longevity and function .
Data Summary
The following table summarizes key findings from various studies on the biological activity of this compound:
Q & A
Basic Research Questions
Q. What established methods ensure high-purity sodium cyanate synthesis in laboratory settings?
- Methodology : this compound is typically synthesized via the oxidation of sodium cyanide using lead oxide or hydrogen peroxide under controlled pH (7–9). Purity is validated through gravimetric analysis and titration . To minimize impurities, recrystallization from aqueous ethanol (70–80% v/v) is recommended. Purity verification should include X-ray diffraction (XRD) for crystallinity and inductively coupled plasma mass spectrometry (ICP-MS) to detect trace metals .
Q. Which spectroscopic techniques are optimal for characterizing this compound, and how should data be interpreted?
- Methodology :
- FTIR : The asymmetric stretching vibration of the NCO⁻ group appears at ~2150–2200 cm⁻¹. Absence of peaks at ~3350 cm⁻¹ (NH stretching) confirms the absence of cyanide impurities .
- NMR : ¹³C NMR shows a resonance at ~120–125 ppm for the cyanate carbon. Compare with reference spectra to confirm structure .
- XRD : Match diffraction patterns with ICSD database entry 24682 for orthorhombic this compound .
- Data Table :
Technique | Key Peaks/Features | Common Artifacts |
---|---|---|
FTIR | 2150–2200 cm⁻¹ | Moisture (broad ~3450 cm⁻¹) |
¹³C NMR | 120–125 ppm | Solvent residues (e.g., DMSO at 40 ppm) |
Q. What safety protocols are critical when handling this compound?
- Methodology : Use fume hoods for powder handling to avoid inhalation. Store in airtight containers with desiccants to prevent hydrolysis to ammonia and carbon dioxide. Emergency protocols should include 0.1% thiocyanate solution for skin exposure and immediate medical consultation for ingestion .
Advanced Research Questions
Q. How can contradictions in reported thermodynamic properties (e.g., ΔfH°) of this compound be resolved?
- Methodology : Discrepancies often arise from synthesis impurities or measurement techniques. Conduct meta-analysis of literature data (e.g., using Web of Science or Scopus ), prioritizing studies with XRD-validated purity. Reproduce key experiments under controlled conditions (e.g., calorimetry in inert atmosphere) and apply error-propagation models to assess uncertainty .
Q. What strategies optimize this compound’s reactivity in novel synthetic pathways (e.g., urea derivatives)?
- Methodology :
- Kinetic Studies : Use stopped-flow spectroscopy to monitor reaction rates with alkyl halides under varying temperatures (25–60°C).
- Solvent Effects : Test polar aprotic solvents (e.g., DMF) to enhance nucleophilicity of NCO⁻.
- Catalysis : Screen transition-metal catalysts (e.g., Cu²⁺) to lower activation barriers. Document yield and byproducts via HPLC .
Q. How to design experiments investigating this compound’s behavior under extreme conditions (e.g., high pH or temperature)?
- Methodology :
- High-Temperature Stability : Use thermogravimetric analysis (TGA) with a ramp rate of 10°C/min under nitrogen. Monitor decomposition onset (>250°C indicates stability) .
- pH-Dependent Hydrolysis : Conduct kinetic studies in buffered solutions (pH 2–12) with ion chromatography to quantify NH₄⁺ and CO₃²⁻ formation .
- Computational Modeling : Validate experimental data with DFT calculations (e.g., Gaussian 16) to predict degradation pathways .
Q. Data Contradiction & Reproducibility
Q. How should researchers address non-reproducible results in this compound-mediated reactions?
- Methodology :
- Control Experiments : Replicate studies using identical reagents (same CAS sources) and equipment calibration.
- Error Analysis : Quantify uncertainties in stoichiometry, mixing efficiency, and ambient humidity .
- Collaborative Trials : Partner with independent labs for interlaboratory validation, sharing raw data via platforms like Zenodo .
Q. Interdisciplinary Applications
Q. What interdisciplinary approaches leverage this compound’s properties (e.g., in biochemistry or materials science)?
- Methodology :
- Biochemical Studies : Use sodium azide as a stable analog (per CynR binding assays ) to explore cyanate’s role in microbial pathways.
- Materials Science : Incorporate this compound into polymer matrices (e.g., epoxy resins) and test thermal stability via DSC .
Q. Literature & Hypothesis Development
Q. How to integrate prior literature into hypothesis-driven this compound research?
Properties
CAS No. |
917-61-3 |
---|---|
Molecular Formula |
CHNNaO |
Molecular Weight |
66.015 g/mol |
IUPAC Name |
sodium;cyanate |
InChI |
InChI=1S/CHNO.Na/c2-1-3;/h3H; |
InChI Key |
VXCSPBPIGBJXJR-UHFFFAOYSA-N |
SMILES |
C(#N)[O-].[Na+] |
Isomeric SMILES |
C(#N)[O-].[Na+] |
Canonical SMILES |
C(#N)O.[Na] |
Key on ui other cas no. |
917-61-3 |
Pictograms |
Irritant |
Synonyms |
CyanicAcid, Sodium Salt ; Sodium Cyanate; Sodium Cyanate (NaOCN); Sodium Isocyanate; Zassol |
Origin of Product |
United States |
Retrosynthesis Analysis
AI-Powered Synthesis Planning: Our tool employs the Template_relevance Pistachio, Template_relevance Bkms_metabolic, Template_relevance Pistachio_ringbreaker, Template_relevance Reaxys, Template_relevance Reaxys_biocatalysis model, leveraging a vast database of chemical reactions to predict feasible synthetic routes.
One-Step Synthesis Focus: Specifically designed for one-step synthesis, it provides concise and direct routes for your target compounds, streamlining the synthesis process.
Accurate Predictions: Utilizing the extensive PISTACHIO, BKMS_METABOLIC, PISTACHIO_RINGBREAKER, REAXYS, REAXYS_BIOCATALYSIS database, our tool offers high-accuracy predictions, reflecting the latest in chemical research and data.
Strategy Settings
Precursor scoring | Relevance Heuristic |
---|---|
Min. plausibility | 0.01 |
Model | Template_relevance |
Template Set | Pistachio/Bkms_metabolic/Pistachio_ringbreaker/Reaxys/Reaxys_biocatalysis |
Top-N result to add to graph | 6 |
Feasible Synthetic Routes
Disclaimer and Information on In-Vitro Research Products
Please be aware that all articles and product information presented on BenchChem are intended solely for informational purposes. The products available for purchase on BenchChem are specifically designed for in-vitro studies, which are conducted outside of living organisms. In-vitro studies, derived from the Latin term "in glass," involve experiments performed in controlled laboratory settings using cells or tissues. It is important to note that these products are not categorized as medicines or drugs, and they have not received approval from the FDA for the prevention, treatment, or cure of any medical condition, ailment, or disease. We must emphasize that any form of bodily introduction of these products into humans or animals is strictly prohibited by law. It is essential to adhere to these guidelines to ensure compliance with legal and ethical standards in research and experimentation.