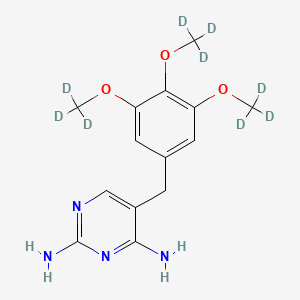
Trimethoprim-d9 (Major)
Overview
Description
Trimethoprim-d9 (Major) is a deuterated analog of Trimethoprim, a bacteriostatic antibiotic that inhibits bacterial dihydrofolate reductase (DHFR). The deuterium substitution occurs at nine hydrogen positions, primarily on the three methoxy groups (-OCH₃ → -OCD₃) and the methylene bridge (CH₂ → CD₂) . This isotopic labeling enhances its utility as an internal standard (IS) in liquid chromatography-tandem mass spectrometry (LC-MS/MS) for quantifying Trimethoprim and related compounds in pharmacokinetic, environmental, and food safety studies . Key properties include:
Mechanism of Action
Target of Action
Trimethoprim-d9, also known as Trimethoprim-d9 (Major), primarily targets the bacterial enzyme dihydrofolate reductase (DHFR) . DHFR is a critical enzyme that catalyzes the formation of tetrahydrofolic acid (THF), an essential precursor in the biosynthesis of nucleic acids .
Mode of Action
Trimethoprim-d9 inhibits DHFR, thereby preventing the synthesis of bacterial DNA and ultimately leading to bacterial death . It binds with a much stronger affinity to bacterial DHFR compared to its mammalian counterpart, allowing Trimethoprim-d9 to selectively interfere with bacterial biosynthetic processes .
Biochemical Pathways
The inhibition of DHFR by Trimethoprim-d9 disrupts the biosynthesis pathways of thymidylate and purines, as well as several other amino acids like glycine, methionine, serine, and N-formyl-methionyl tRNA . This leads to an imbalance in the pathways involved in active synthesizing thymidylate, disrupts DNA replication, and eventually causes cell death .
Pharmacokinetics
Trimethoprim-d9 is a potent inhibitor of multidrug and toxin extrusion protein (MATE) and a weak inhibitor of cytochrome P450 (CYP) 2C8 . These properties can influence the absorption, distribution, metabolism, and excretion (ADME) of the compound, impacting its bioavailability .
Result of Action
The molecular and cellular effects of Trimethoprim-d9’s action include the inhibition of bacterial DNA synthesis, leading to bacterial death . Some of the new analogs of Trimethoprim-d9 inhibited DHFR activity more strongly than Trimethoprim did, indicating that the addition of amide bonds into the analogs of Trimethoprim-d9 increases their affinity towards DHFR .
Action Environment
Environmental factors can influence the action, efficacy, and stability of Trimethoprim-d9. For instance, pH plays a role in the mode of action of Trimethoprim-d9 on Escherichia coli . Moreover, soil-related factors, animal husbandry and waste management, potable and wastewater, and food safety can contribute to antimicrobial resistance . These factors need to be considered in modeling the fate and transport of Trimethoprim-d9 in coastal/estuarine waters .
Biochemical Analysis
Biochemical Properties
Trimethoprim-d9 interacts with the enzyme dihydrofolate reductase (DHFR), which plays a crucial role in the biosynthesis pathways of thymidylate, purines, and several amino acids . The interaction between Trimethoprim-d9 and DHFR inhibits the enzyme’s activity, disrupting DNA replication and eventually leading to cell death .
Cellular Effects
The effects of Trimethoprim-d9 on cells are primarily due to its inhibition of DHFR. This disruption in folate metabolism leads to an imbalance in the pathways involved in synthesizing thymidylate, which is essential for DNA replication . As a result, the function of cells is significantly affected, leading to cell death .
Molecular Mechanism
Trimethoprim-d9 exerts its effects at the molecular level by binding to the DHFR enzyme. This binding inhibits the enzyme’s activity, preventing the reduction of dihydrofolate acid to tetrahydrofolic acid . This disruption in folate metabolism leads to an imbalance in the pathways involved in synthesizing thymidylate, which is essential for DNA replication .
Metabolic Pathways
Trimethoprim-d9 is involved in the folate metabolism pathway. It interacts with the DHFR enzyme, which catalyzes the reduction of dihydrofolate acid to tetrahydrofolic acid . The inhibition of DHFR by Trimethoprim-d9 disrupts this metabolic pathway, leading to an imbalance in the pathways involved in synthesizing thymidylate .
Biological Activity
Trimethoprim-d9 (Major) is a deuterated analog of trimethoprim, a well-known antibiotic primarily used for treating bacterial infections. The deuteration enhances its tracking in biological studies, providing insights into its pharmacokinetics and metabolic pathways. This article explores the biological activity of Trimethoprim-d9, focusing on its mechanism of action, efficacy against various pathogens, and relevant research findings.
Trimethoprim-d9 functions as a reversible inhibitor of dihydrofolate reductase (DHFR), an enzyme essential for synthesizing tetrahydrofolic acid (THF) from dihydrofolate (DHF). This inhibition disrupts the production of nucleic acids and proteins in bacteria, leading to their growth inhibition and eventual cell death.
- Selectivity : Trimethoprim-d9 exhibits a higher affinity for bacterial DHFR compared to mammalian DHFR, which allows it to selectively target bacterial biosynthetic processes without significantly affecting human cells .
Efficacy Against Bacterial Strains
Trimethoprim-d9 has shown effectiveness against a range of bacterial pathogens. Its performance can be evaluated through various assays:
Pathogen | Efficacy (IC50) | Reference |
---|---|---|
Escherichia coli | 55.26 µM | |
Staphylococcus aureus | 10.5 µM | |
Klebsiella pneumoniae | 30 µM |
The IC50 values indicate the concentration required to inhibit 50% of the bacterial growth, showcasing Trimethoprim-d9's potential as an effective antibacterial agent.
Pharmacokinetics
The pharmacokinetic profile of Trimethoprim-d9 is similar to that of its parent compound:
- Absorption : Achieves peak serum concentrations within 1-4 hours post-administration.
- Distribution : Extensively distributed in body tissues, including sputum and vaginal fluids.
- Metabolism : Primarily metabolized by CYP enzymes, with about 10-20% excreted as metabolites .
Case Studies and Research Findings
Several studies have explored the biological activity and potential applications of Trimethoprim-d9:
- Inhibition Studies : A study demonstrated that Trimethoprim-d9 effectively inhibits DHFR in various bacterial strains, with some analogs showing improved potency compared to traditional trimethoprim .
- Resistance Mechanisms : Research indicates that Trimethoprim-d9 can be utilized to study bacterial resistance mechanisms due to its selective inhibition profile. This is crucial for developing new strategies to combat antibiotic resistance .
- Combination Therapy : Trimethoprim-d9 is often studied in combination with sulfamethoxazole, which inhibits an earlier step in folate synthesis, leading to synergistic effects against resistant bacterial strains .
Scientific Research Applications
Pharmacokinetic Studies
Trimethoprim-D9 is primarily utilized as an internal standard in pharmacokinetic studies to quantify the concentration of trimethoprim and its metabolites in biological samples. The deuterated form allows for precise measurement due to its distinct mass, facilitating the differentiation from non-deuterated compounds during mass spectrometry analysis.
Table 1: Pharmacokinetic Parameters of Trimethoprim-D9
Parameter | Value |
---|---|
Cmax (µg/ml) | 2.1 ± 1.0 |
Tmax (h) | 1.5 |
Half-life (h) | 0.88 |
Elimination rate (1/h) | 0.0093 ± 0.0011 |
AUCt (µg.h/ml) | 2.901 ± 1.4 |
Volume of distribution (l/kg) | 2.632 |
Clearance (l/h) | 2.7 |
This data is crucial for understanding the pharmacokinetics of trimethoprim in various populations, including pediatric patients and those with renal impairment .
Clinical Applications
Trimethoprim-D9 has been employed in clinical settings to evaluate the efficacy and safety of trimethoprim-sulfamethoxazole combinations against infections such as Pneumocystis jirovecii pneumonia, particularly in immunocompromised patients.
Case Study: Treatment of Pneumocystis Jirovecii Pneumonia
A notable case involved a lymphoma patient who developed Pneumocystis jirovecii pneumonia after chemotherapy. The patient was treated with a regimen that included trimethoprim-sulfamethoxazole, leading to significant improvement in respiratory symptoms and resolution of pulmonary infiltrates as observed on follow-up imaging .
Toxicological Assessments
The use of Trimethoprim-D9 extends to toxicological studies where it serves as a reference standard for assessing the safety profiles of trimethoprim and its metabolites. Research indicates that certain metabolites can form reactive protein adducts, which are crucial for understanding adverse drug reactions .
Table 2: Toxicological Findings Related to Trimethoprim Metabolites
Metabolite | Toxicity Observed |
---|---|
Sulfamethoxazole-N-acetyl | Potential nephrotoxicity |
Trimethoprim | Rare cases of hematologic toxicity |
These findings underscore the importance of monitoring drug levels in patients to mitigate risks associated with elevated concentrations of these compounds .
Method Development for Analysis
Recent advancements have led to the development of robust analytical methods using liquid chromatography-tandem mass spectrometry (LC-MS/MS) for the simultaneous quantification of trimethoprim and sulfamethoxazole in serum and plasma samples.
Table 3: Analytical Method Validation Parameters
Parameter | Result |
---|---|
Linearity | R² > 0.99 |
Precision (CV %) | < 5% |
Recovery (%) | 90-110% |
Stability | Up to 120 hours |
This method has been validated according to FDA guidelines, ensuring its applicability for routine therapeutic drug monitoring and clinical studies focused on optimizing treatment regimens for various infectious diseases .
Q & A
Q. Basic: What analytical techniques are essential for characterizing Trimethoprim-d9 to confirm isotopic purity and structural integrity?
Answer:
Trimethoprim-d9 requires rigorous characterization using:
- Mass Spectrometry (MS): High-resolution MS (HRMS) quantifies the molecular ion ([M+H]+) at m/z 299.194 (exact mass) to confirm isotopic enrichment (nine deuterium atoms) and rule out impurities .
- Nuclear Magnetic Resonance (NMR): ¹H NMR detects residual proton signals in deuterated positions, while ¹³C NMR verifies the integrity of the trimethoxybenzyl-pyrimidine backbone .
- Isotopic Purity Assessment: Use liquid chromatography (LC) coupled with UV detection to separate Trimethoprim-d9 from non-deuterated analogs, ensuring ≥98% isotopic purity .
Q. Basic: How should Trimethoprim-d9 be stored to maintain stability in long-term studies?
Answer:
- Temperature: Store at -20°C to prevent thermal degradation, as the compound is temperature-sensitive .
- Solvent Compatibility: Dissolve in deuterated solvents (e.g., DMSO-d6) for NMR studies or methanol for LC-MS workflows to avoid solvent-induced degradation.
- Light Exposure: Use amber vials to block UV light, which can destabilize the pyrimidine ring .
- Documentation: Track storage conditions in metadata to correlate stability with experimental outcomes, following NIH preclinical reporting guidelines .
Q. Advanced: How can researchers design pharmacokinetic (PK) studies using Trimethoprim-d9 as an internal standard?
Answer:
Experimental Design Considerations:
- Dose Calibration: Co-administer Trimethoprim-d9 with the non-deuterated drug in preclinical models (e.g., rodents) to normalize extraction efficiency and matrix effects during LC-MS/MS analysis .
- Sampling Timepoints: Collect plasma/tissue samples at 0.5, 1, 2, 4, 8, and 24 hours post-dose to capture absorption/distribution phases.
- Control Groups: Include cohorts treated with deuterated and non-deuterated compounds to isolate isotopic effects on PK parameters (e.g., half-life, volume of distribution) .
Data Validation: Use a two-way ANOVA to compare inter-day variability in deuterated vs. non-deuterated groups, with p < 0.05 indicating significance .
Q. Advanced: How to resolve discrepancies in reported isotopic purity values across studies?
Answer:
Methodological Steps:
Replicate Conditions: Reproduce synthesis/purification protocols from conflicting studies (e.g., reaction time, deuterium source) .
Cross-Validate Techniques: Combine LC-MS (quantitative) with ¹H NMR (qualitative) to detect low-abundance non-deuterated impurities .
Statistical Harmonization: Apply the FINER framework to evaluate study feasibility, novelty, and ethical rigor, identifying outliers in methodology or instrumentation .
Meta-Analysis: Pool data from ≥5 independent studies using random-effects models to calculate weighted mean purity values, reporting 95% confidence intervals .
Q. Advanced: What strategies optimize LC-MS/MS detection of Trimethoprim-d9 in complex biological matrices?
Answer:
Optimization Workflow:
- Ionization Parameters: Use electrospray ionization (ESI+) with capillary voltage = 3.5 kV and desolvation temperature = 450°C to enhance ionizability of the deuterated compound .
- Matrix Interference Mitigation: Apply solid-phase extraction (SPE) with C18 cartridges to remove lipids/proteins from plasma samples.
- Collision Energy Calibration: Test energies between 15–35 eV to maximize fragmentation of Trimethoprim-d9 (e.g., m/z 299→230 transition) while minimizing background noise .
Validation: Perform spike-and-recovery experiments in triplicate across three days, accepting ≤15% coefficient of variation (CV) .
Q. Advanced: How to statistically account for isotopic effects in Trimethoprim-d9-based assays?
Answer:
- Standard Curve Normalization: Prepare calibration curves with Trimethoprim-d9 in the same matrix as test samples to correct for deuterium-induced retention time shifts .
- Multivariate Regression: Include isotopic purity (%D) as a covariate in pharmacokinetic models to quantify its impact on clearance rates .
- Error Propagation Analysis: Calculate combined uncertainty (e.g., ±0.5% for purity, ±2% for instrument error) using the Guide to the Expression of Uncertainty in Measurement (GUM) .
Comparison with Similar Compounds
Trimethoprim-d9 (Major) is compared below with structurally related deuterated analogs and non-deuterated Trimethoprim.
Trimethoprim (Non-deuterated)
- CAS Number : 738-70-5
- Molecular Formula : C₁₄H₁₈N₄O₃
- Molecular Weight : 290.32 g/mol
- Key Differences: Lacks deuterium substitution, leading to a lower molecular weight (~3% difference). Used therapeutically for urinary tract infections and Pneumocystis pneumonia, whereas Trimethoprim-d9 is exclusively for analytical purposes . In LC-MS, unlabeled Trimethoprim cannot serve as an IS due to interference with target analytes .
Trimethoprim-d3
- CAS Number: Not explicitly listed (see ).
- Molecular Formula : C₁₄H₁₅D₃N₄O₃
- Molecular Weight : 293.34 g/mol .
- Key Differences: Contains three deuterium atoms, likely on the methylene bridge (CD₂) rather than methoxy groups. Lower isotopic enrichment (D3 vs. D9) reduces mass shift in MS, increasing risk of spectral overlap with non-deuterated analogs .
Trimethoprim-13C3
- CAS Number: Not explicitly listed.
- Molecular Formula : C₁₁¹³C₃H₁₈N₄O₃
- Molecular Weight : 293.30 g/mol .
- Key Differences :
Analytical Performance in Research Studies
Detection Frequency and Sensitivity
- Trimethoprim-d9 demonstrated 100% detection frequency in 22 samples (urine, dust, food) with intensities of 10⁶–10⁹ in positive-ion MS mode, outperforming other IS like paroxetine-d4 (23%) .
- Trimethoprim-d3 and Trimethoprim-13C3 are less commonly used due to lower isotopic enrichment and smaller mass shifts .
Interference and Specificity
- Misuse of non-deuterated Trimethoprim as an IS caused false-positive results in proficiency testing, highlighting the necessity of Trimethoprim-d9’s distinct isotopic signature .
- Trimethoprim-d9’s +9 Da mass shift minimizes overlap with endogenous compounds and co-eluting analytes .
Research Findings and Limitations
- Advantages of Trimethoprim-d9 :
- Limitations: Limited commercial availability compared to non-deuterated Trimethoprim . Ecological and toxicological data (e.g., biodegradation, bioaccumulation) remain unstudied .
Properties
IUPAC Name |
5-[[3,4,5-tris(trideuteriomethoxy)phenyl]methyl]pyrimidine-2,4-diamine | |
---|---|---|
Source | PubChem | |
URL | https://pubchem.ncbi.nlm.nih.gov | |
Description | Data deposited in or computed by PubChem | |
InChI |
InChI=1S/C14H18N4O3/c1-19-10-5-8(6-11(20-2)12(10)21-3)4-9-7-17-14(16)18-13(9)15/h5-7H,4H2,1-3H3,(H4,15,16,17,18)/i1D3,2D3,3D3 | |
Source | PubChem | |
URL | https://pubchem.ncbi.nlm.nih.gov | |
Description | Data deposited in or computed by PubChem | |
InChI Key |
IEDVJHCEMCRBQM-GQALSZNTSA-N | |
Source | PubChem | |
URL | https://pubchem.ncbi.nlm.nih.gov | |
Description | Data deposited in or computed by PubChem | |
Canonical SMILES |
COC1=CC(=CC(=C1OC)OC)CC2=CN=C(N=C2N)N | |
Source | PubChem | |
URL | https://pubchem.ncbi.nlm.nih.gov | |
Description | Data deposited in or computed by PubChem | |
Isomeric SMILES |
[2H]C([2H])([2H])OC1=CC(=CC(=C1OC([2H])([2H])[2H])OC([2H])([2H])[2H])CC2=CN=C(N=C2N)N | |
Source | PubChem | |
URL | https://pubchem.ncbi.nlm.nih.gov | |
Description | Data deposited in or computed by PubChem | |
Molecular Formula |
C14H18N4O3 | |
Source | PubChem | |
URL | https://pubchem.ncbi.nlm.nih.gov | |
Description | Data deposited in or computed by PubChem | |
DSSTOX Substance ID |
DTXSID10662219 | |
Record name | Trimethoprim-d9 | |
Source | EPA DSSTox | |
URL | https://comptox.epa.gov/dashboard/DTXSID10662219 | |
Description | DSSTox provides a high quality public chemistry resource for supporting improved predictive toxicology. | |
Molecular Weight |
299.37 g/mol | |
Source | PubChem | |
URL | https://pubchem.ncbi.nlm.nih.gov | |
Description | Data deposited in or computed by PubChem | |
CAS No. |
1189460-62-5 | |
Record name | Trimethoprim-d9 | |
Source | EPA DSSTox | |
URL | https://comptox.epa.gov/dashboard/DTXSID10662219 | |
Description | DSSTox provides a high quality public chemistry resource for supporting improved predictive toxicology. | |
Synthesis routes and methods I
Procedure details
Synthesis routes and methods II
Procedure details
Disclaimer and Information on In-Vitro Research Products
Please be aware that all articles and product information presented on BenchChem are intended solely for informational purposes. The products available for purchase on BenchChem are specifically designed for in-vitro studies, which are conducted outside of living organisms. In-vitro studies, derived from the Latin term "in glass," involve experiments performed in controlled laboratory settings using cells or tissues. It is important to note that these products are not categorized as medicines or drugs, and they have not received approval from the FDA for the prevention, treatment, or cure of any medical condition, ailment, or disease. We must emphasize that any form of bodily introduction of these products into humans or animals is strictly prohibited by law. It is essential to adhere to these guidelines to ensure compliance with legal and ethical standards in research and experimentation.