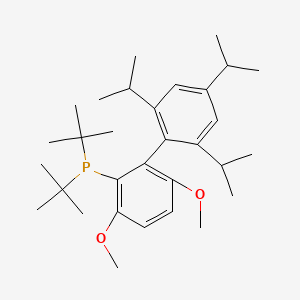
tBuBrettPhos
Overview
Description
tBuBrettPhos (CAS 1160861-53-9) is a bulky, electron-rich bisphosphine ligand developed by the Buchwald group for palladium-catalyzed cross-coupling reactions. Its chemical formula is C₃₁H₄₉O₂P, with a molecular weight of 484.69 g/mol . Structurally, it features a biaryl backbone substituted with tert-butyl groups and methoxy substituents, which confer exceptional steric bulk and thermal stability. This ligand is a white crystalline solid, air- and moisture-stable, and compatible with diverse reaction conditions .
Mechanism of Action
Target of Action
tBuBrettPhos is a dialkylbiaryl phosphine ligand . It is primarily targeted towards palladium , a transition metal, and is widely used in palladium-catalyzed cross-coupling reactions .
Mode of Action
This compound interacts with its target, palladium, to form a complex that acts as a precatalyst . This precatalyst promotes cross-coupling reactions more efficiently and exhibits improved reactivity compared to other catalytic systems . The formation of the active catalytic species is efficient, and the ligand to palladium ratio can be accurately controlled .
Biochemical Pathways
The primary biochemical pathway influenced by this compound involves the formation of C-C, C–N, C–O, C–F, C–CF3, and C–S bonds . These bonds are crucial in the synthesis of various organic compounds. The compound’s action can affect multiple downstream effects, including the synthesis of complex organic molecules.
Pharmacokinetics
Its solubility in a wide range of common organic solvents suggests that it could have good distribution properties if used in a biological context.
Result of Action
The result of this compound’s action is the efficient promotion of cross-coupling reactions . This leads to the formation of various organic compounds, including those with C-C, C–N, C–O, C–F, C–CF3, and C–S bonds . It has been used as a precatalyst for the N-arylation of amino acid esters with aryl triflates under mild reaction conditions and minimal racemization of the amino acid ester .
Action Environment
This compound is air-, moisture-, and thermally-stable , suggesting that it can maintain its efficacy and stability under a variety of environmental conditions.
Biological Activity
tBuBrettPhos, a phosphine ligand, has garnered attention in the field of organometallic chemistry due to its significant role in catalysis, particularly in palladium-catalyzed reactions. This article explores the biological activity of this compound, focusing on its applications, mechanisms of action, and relevant research findings.
Chemical Structure and Properties
This compound is characterized by a bulky tert-butyl group that enhances its steric properties, making it a valuable ligand in various catalytic processes. Its structure allows for effective coordination with transition metals, facilitating reactions such as cross-coupling and C–S bond formation.
Biological Activity Overview
While this compound is primarily recognized for its catalytic applications, recent studies have begun to explore its biological implications. The biological activity can be categorized into several key areas:
- Catalytic Efficiency : this compound has shown high yields in palladium-catalyzed reactions, which are crucial in synthesizing biologically active compounds.
- Inhibition Studies : Research indicates potential inhibitory effects on certain biological pathways, although specific studies on direct biological targets remain limited.
1. Catalytic Applications
This compound has been extensively studied for its role in catalyzing C–S cross-coupling reactions. A study demonstrated that using this compound as a ligand resulted in yields exceeding 97% when applied to aryl thiol arylation reactions (Table 1) .
Reaction Type | Yield (%) | Conditions |
---|---|---|
Aryl Thiol Arylation | 97% | Room temperature, 2 hours |
C–N Cross-Coupling | 91% | Varying aryl halides |
2. Inhibition of CYP Enzymes
A study evaluated the inhibition potential of various phosphine ligands, including this compound, on cytochrome P450 enzymes (CYPs). While specific IC50 values for this compound were not reported, the study highlighted its role in modulating enzyme activity indirectly through catalytic pathways .
3. Mechanistic Insights
Research into the mechanistic aspects of this compound revealed that it enhances the stability of palladium catalysts under various reaction conditions. This stability is crucial for maintaining enzyme functionality during catalytic processes .
Discussion
The biological activity of this compound is primarily linked to its efficacy as a ligand in catalysis rather than direct biological interactions. Its ability to facilitate high-yield reactions makes it an essential compound in synthesizing pharmaceuticals and other biologically relevant molecules. However, more focused research is needed to elucidate any direct biological effects or interactions with specific biological targets.
Q & A
Basic Research Questions
Q. What are the standard reaction conditions for employing tBuBrettPhos in palladium-catalyzed cross-coupling reactions?
- Methodological Answer : Optimal conditions include:
- Concentration : 11 μM (1.1 equivalents) of this compound .
- Solvent System : 50 mM HEPES buffer (pH 8.5) with a 95:5 water/DMSO ratio to balance solubility and reactivity .
- Temperature : 37°C for 24 hours, though room-temperature applications are feasible with electron-neutral aryl bromides .
- Catalyst System : Pd complexes ligated with this compound, which stabilize oxidative addition intermediates .
Q. How is this compound synthesized, and what analytical methods confirm its purity and structure?
- Methodological Answer :
- Synthesis : this compound is prepared via sequential functionalization of a phosphine core, incorporating tert-butyl groups for steric bulk and a bromine substituent for reactivity .
- Characterization : Use / NMR spectroscopy to confirm ligand identity and purity. Mass spectrometry (e.g., ESI-MS) is critical for verifying molecular weight, particularly in post-reaction analyses .
Q. Why is this compound preferred over other phosphine ligands in protein cross-linking studies?
- Methodological Answer :
- Steric and Electronic Profile : Its bulky tert-butyl groups prevent catalyst deactivation, while the bromine atom facilitates covalent bonding with target proteins like SrtA .
- Compatibility : Works in aqueous buffers (e.g., HEPES) at physiological temperatures, making it suitable for biomolecular applications .
Advanced Research Questions
Q. How can conflicting mass spectrometry data be resolved when this compound-mediated reactions yield unexpected cross-linking products?
- Methodological Answer :
- Step 1 : Compare pre- and post-reaction mass spectra to identify adducts or incomplete reactions. For example, unreacted SrtA (~18 kDa) vs. crosslinked SrtA* (~36 kDa) .
- Step 2 : Conduct control experiments without Pd catalysts to rule out non-specific interactions.
- Step 3 : Use tandem MS (MS/MS) to fragment ambiguous peaks and confirm covalent bond formation .
Q. What strategies optimize this compound for C-O coupling reactions at sub-ambient temperatures?
- Methodological Answer :
- Ligand Pairing : Combine this compound with Pd precursors like Pd(OAc) to enhance catalytic activity at 25°C .
- Substrate Tuning : Prioritize electron-neutral aryl bromides, which undergo oxidative addition more readily under mild conditions .
- Solvent Optimization : Reduce DMSO content (<5%) to minimize competing side reactions while maintaining ligand solubility .
Q. How do mechanistic studies differentiate this compound from structurally similar ligands (e.g., AdBrettPhos) in suppressing diarylation?
- Methodological Answer :
- Kinetic Studies : Monitor reaction progress via NMR to compare turnover rates. This compound slows secondary arylation due to steric hindrance .
- Computational Modeling : DFT calculations can reveal differences in transition-state stabilization between ligands .
- Competitive Experiments : Perform parallel reactions with AdBrettPhos to quantify diarylation byproducts via HPLC .
Q. What experimental controls are essential when this compound-based reactions exhibit batch-to-batch variability?
- Methodological Answer :
- Control 1 : Include a reference reaction with a standardized substrate (e.g., 4-bromotoluene) to assess catalyst activity.
- Control 2 : Test ligand purity via NMR before each use to rule out oxidation or degradation.
- Control 3 : Monitor Pd:ligand stoichiometry (e.g., 1:1.1 ratio) to ensure catalytic species integrity .
Q. Data Analysis & Reproducibility
Q. How should researchers address discrepancies in reaction yields when replicating this compound-mediated protocols?
- Methodological Answer :
- Variable Screening : Systematically test parameters like buffer pH (8.5–9.0), DMSO purity, and degassing methods (N vs. Ar) .
- Data Normalization : Report yields relative to internal standards (e.g., 1,3,5-trimethoxybenzene) to account for instrumental variability.
- Open Data Practices : Share raw spectra and reaction logs via repositories like Zenodo to enable direct comparisons .
Comparison with Similar Compounds
Catalytic Performance
Data from benchmark studies highlight tBuBrettPhos’s versatility and efficiency relative to other ligands (Table 1):
Table 1: Catalytic Performance in C–N Coupling (Benchmark Data from Summit Framework )
Catalyst | Base | Yield (%) | Cost ($) |
---|---|---|---|
tBuXPhos | DBU | 78 | 0.25 |
This compound | DBU | 90 | 0.28 |
AlPhos | BTMG | 109 | 0.52 |
While AlPhos achieves higher yields, its cost is nearly double that of this compound. This compound strikes a balance between cost and performance, making it preferable for large-scale applications .
Substrate Scope and Selectivity
- Sterically Hindered Substrates : this compound outperforms less bulky ligands (e.g., BrettPhos, XPhos) in reactions involving ortho-substituted aryl bromides, achieving yields >80% .
- Cascade Reactions : In palladium-catalyzed benzimidazole synthesis, this compound enables regioselective transformations unattainable with smaller ligands like SPhos or RuPhos .
- Secondary Amines : Unlike BrettPhos, which favors primary amines, this compound shows broader compatibility with secondary amines in Buchwald-Hartwig couplings .
Cost-Effectiveness
This compound is more cost-effective than AlPhos ($0.28 vs. $0.52 per mmol) and comparable to tBuXPhos ($0.25). In multi-objective optimizations considering both yield and cost, this compound is prioritized for reactions where moderate ligand costs are acceptable for higher yields .
Thermal Stability and Precatalyst Compatibility
This compound forms stable oxidative addition complexes (OACs) with Pd, such as the this compound Pd G6 TES precatalyst. These OACs exhibit superior activity in C–O couplings compared to Pd(OAc)₂/tBuXPhos systems, with TONs exceeding 680 .
Ligand Classification and Electronic Effects
This compound belongs to the aryl-type phosphine ligand family (alongside XPhos, SPhos, and RuPhos), characterized by intermediate ΔEₐd values (~5 kJ/mol) that balance steric bulk and electronic donation. This contrasts with alkyl-type ligands (e.g., P(tBu)₃), which have higher ΔEₐd but lower functional group tolerance .
Key Research Findings
High-Yield Cross-Couplings : this compound/Pd systems achieve >90% yield in Buchwald-Hartwig aminations at low catalyst loadings (0.1 mol% Pd) .
Regioselective Catalysis : In cascade reactions, this compound enables dual C–N and C–O bond formation with complete regiocontrol .
Cost-Driven Optimization : this compound is optimal for nucleophilic aromatic substitutions where ligand cost must remain below $0.30/mmol .
Properties
IUPAC Name |
ditert-butyl-[3,6-dimethoxy-2-[2,4,6-tri(propan-2-yl)phenyl]phenyl]phosphane | |
---|---|---|
Source | PubChem | |
URL | https://pubchem.ncbi.nlm.nih.gov | |
Description | Data deposited in or computed by PubChem | |
InChI |
InChI=1S/C31H49O2P/c1-19(2)22-17-23(20(3)4)27(24(18-22)21(5)6)28-25(32-13)15-16-26(33-14)29(28)34(30(7,8)9)31(10,11)12/h15-21H,1-14H3 | |
Source | PubChem | |
URL | https://pubchem.ncbi.nlm.nih.gov | |
Description | Data deposited in or computed by PubChem | |
InChI Key |
REWLCYPYZCHYSS-UHFFFAOYSA-N | |
Source | PubChem | |
URL | https://pubchem.ncbi.nlm.nih.gov | |
Description | Data deposited in or computed by PubChem | |
Canonical SMILES |
CC(C)C1=CC(=C(C(=C1)C(C)C)C2=C(C=CC(=C2P(C(C)(C)C)C(C)(C)C)OC)OC)C(C)C | |
Source | PubChem | |
URL | https://pubchem.ncbi.nlm.nih.gov | |
Description | Data deposited in or computed by PubChem | |
Molecular Formula |
C31H49O2P | |
Source | PubChem | |
URL | https://pubchem.ncbi.nlm.nih.gov | |
Description | Data deposited in or computed by PubChem | |
DSSTOX Substance ID |
DTXSID70657989 | |
Record name | Di-tert-butyl[3,6-dimethoxy-2',4',6'-tri(propan-2-yl)[1,1'-biphenyl]-2-yl]phosphane | |
Source | EPA DSSTox | |
URL | https://comptox.epa.gov/dashboard/DTXSID70657989 | |
Description | DSSTox provides a high quality public chemistry resource for supporting improved predictive toxicology. | |
Molecular Weight |
484.7 g/mol | |
Source | PubChem | |
URL | https://pubchem.ncbi.nlm.nih.gov | |
Description | Data deposited in or computed by PubChem | |
CAS No. |
1160861-53-9 | |
Record name | Di-tert-butyl[3,6-dimethoxy-2',4',6'-tri(propan-2-yl)[1,1'-biphenyl]-2-yl]phosphane | |
Source | EPA DSSTox | |
URL | https://comptox.epa.gov/dashboard/DTXSID70657989 | |
Description | DSSTox provides a high quality public chemistry resource for supporting improved predictive toxicology. | |
Record name | Di-tert-butyl(2',4',6'-triisopropyl-3,6-dimethoxy-[1,1'-biphenyl]-2-yl)phosphine | |
Source | European Chemicals Agency (ECHA) | |
URL | https://echa.europa.eu/information-on-chemicals | |
Description | The European Chemicals Agency (ECHA) is an agency of the European Union which is the driving force among regulatory authorities in implementing the EU's groundbreaking chemicals legislation for the benefit of human health and the environment as well as for innovation and competitiveness. | |
Explanation | Use of the information, documents and data from the ECHA website is subject to the terms and conditions of this Legal Notice, and subject to other binding limitations provided for under applicable law, the information, documents and data made available on the ECHA website may be reproduced, distributed and/or used, totally or in part, for non-commercial purposes provided that ECHA is acknowledged as the source: "Source: European Chemicals Agency, http://echa.europa.eu/". Such acknowledgement must be included in each copy of the material. ECHA permits and encourages organisations and individuals to create links to the ECHA website under the following cumulative conditions: Links can only be made to webpages that provide a link to the Legal Notice page. | |
Disclaimer and Information on In-Vitro Research Products
Please be aware that all articles and product information presented on BenchChem are intended solely for informational purposes. The products available for purchase on BenchChem are specifically designed for in-vitro studies, which are conducted outside of living organisms. In-vitro studies, derived from the Latin term "in glass," involve experiments performed in controlled laboratory settings using cells or tissues. It is important to note that these products are not categorized as medicines or drugs, and they have not received approval from the FDA for the prevention, treatment, or cure of any medical condition, ailment, or disease. We must emphasize that any form of bodily introduction of these products into humans or animals is strictly prohibited by law. It is essential to adhere to these guidelines to ensure compliance with legal and ethical standards in research and experimentation.