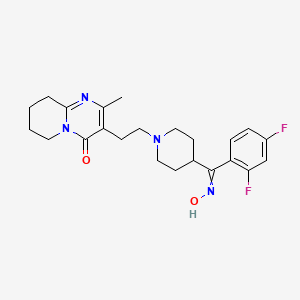
Risperidone E-Oxime
Overview
Description
Risperidone E-Oxime (CAS: 691007-09-7; molecular formula: C₂₃H₂₈F₂N₄O₂) is an impurity identified in commercial preparations of risperidone, a second-generation antipsychotic used to treat schizophrenia and related psychotic disorders . It is a stereoisomer of Risperidone Z-Oxime (CAS: 132961-05-8), differing in the spatial configuration of the oxime group (-NOH) attached to the difluorophenyl ring . As a pharmacopeial impurity, its presence in risperidone formulations is tightly regulated to ensure drug safety and efficacy. While this compound itself lacks therapeutic activity, its structural similarity to risperidone underscores the importance of monitoring impurities during drug manufacturing and storage .
Preparation Methods
Synthetic Routes and Reaction Conditions
The synthesis of Risperidone E-Oxime typically involves multiple steps, including the formation of the piperidine ring, the introduction of the difluorophenyl group, and the construction of the tetrahydropyridopyrimidinone core. Specific reaction conditions, such as temperature, pressure, and the use of catalysts, are crucial for the successful synthesis of this compound.
Industrial Production Methods
Industrial production methods for this compound may involve large-scale synthesis techniques, including continuous flow reactors and automated synthesis systems. These methods aim to optimize yield, purity, and cost-effectiveness while ensuring safety and environmental compliance.
Chemical Reactions Analysis
Cyclization Reactions
-
Z-isomer oxime readily undergoes base-mediated cyclization (e.g., using KOH or NaOH) to form the benzisoxazole core of risperidone .
-
E-Oxime , by contrast, is kinetically inert under identical conditions due to its anti-configuration, which sterically hinders nucleophilic attack on the carbonyl carbon .
Key Mechanistic Steps for Z-Oxime Cyclization :
-
Nucleophilic attack : The oxime oxygen attacks the adjacent carbonyl carbon.
-
C–O bond cleavage : Facilitated by base, forming the benzisoxazole ring.
-
Proton transfer : Stabilizes the aromatic system.
Alkylation Reactions
Alkylation of the piperidine nitrogen is another pivotal reaction in risperidone synthesis. Both Z- and E-oximes participate, but outcomes differ:
-
Z-Oxime : Reacts with 3-(2-chloroethyl)-6,7,8,9-tetrahydro-2-methyl-4H-pyrido[1,2-a]pyrimidin-4-one (5 ) in acetonitrile under reflux with K₂CO₃, yielding alkylated Z-oxime (7 ) without isomerization .
-
E-Oxime : Similarly undergoes alkylation but retains its configuration, leading to alkylated E-oxime derivatives that remain unreactive in subsequent cyclization steps .
Reaction Conditions Table:
Parameter | Details |
---|---|
Solvent | Acetonitrile |
Base | K₂CO₃ |
Temperature | Reflux (~80°C) |
Time | 3–5 hours |
Yield (Z-isomer) | >95% |
Isomerization and Stability
Risperidone E-Oxime does not isomerize to the Z-form under standard synthetic conditions, which explains its persistence as an impurity . Key factors influencing stability:
-
Thermodynamic preference : The Z-isomer is energetically favored due to intramolecular hydrogen bonding between the oxime hydroxyl and fluorine atoms .
-
Acid/Base Effects : Protonation in acetic acid promotes Z-isomer precipitation as a salt, enabling separation from E-Oxime .
Comparative Reactivity of Z- vs. E-Oxime
Property | Z-Oxime | E-Oxime |
---|---|---|
Cyclization Activity | High (forms benzisoxazole) | None |
Alkylation | Proceeds without isomerization | Proceeds without isomerization |
Stability in Acid | Precipitates as acetate salt | Remains in solution |
Synthetic Utility | Key intermediate for risperidone | Process impurity |
Analytical Detection and Implications
Scientific Research Applications
Chemistry
In chemistry, this compound can be used as a building block for the synthesis of more complex molecules. Its unique structure allows for the exploration of new chemical reactions and pathways.
Biology
In biological research, this compound may be used to study its interactions with various biological targets, such as enzymes and receptors. Its potential as a biochemical probe can provide insights into cellular processes and mechanisms.
Medicine
In medicine, Risperidone E-Oxime may have therapeutic potential due to its ability to interact with specific molecular targets. Research into its pharmacological properties could lead to the development of new drugs.
Industry
In industry, this compound can be used in the development of new materials, such as polymers and coatings. Its unique chemical properties may enhance the performance and durability of these materials.
Mechanism of Action
The mechanism of action of Risperidone E-Oxime involves its interaction with specific molecular targets, such as enzymes or receptors. These interactions can modulate various biochemical pathways, leading to the compound’s observed effects. The exact molecular targets and pathways involved depend on the specific application and context of use.
Comparison with Similar Compounds
Structural and Pharmacological Comparisons with Other Risperidone Impurities
Risperidone E-Oxime belongs to a class of oxidative metabolites and synthetic byproducts of risperidone. Key impurities include:
Key Findings :
- 9-Hydroxyrisperidone, unlike E-Oxime, is pharmacologically active and contributes to risperidone’s efficacy via CYP2D6-mediated metabolism .
Comparison with Structurally Related Antipsychotics
Risperidone and its analogs share structural motifs with other antipsychotics, influencing receptor binding and metabolic profiles:
2.2.1. Ketanserin and Ziprasidone
- Structural Similarity: Risperidone and ketanserin both feature a benzisoxazole core but differ in substituents. Ketanserin has a quinoline group, while risperidone includes a fluorophenyl-piperidine moiety . Ziprasidone shares a piperazine ring with risperidone but incorporates a benzisothiazole group .
- Receptor Affinity: Compound 5-HT₂A Affinity D₂ Affinity Similarity Score vs. Risperidone Risperidone High Moderate Reference (1.00) Ketanserin High Low 0.85 (8-quinoline series) Ziprasidone High Moderate 0.92 (6-quinoline series)
Clinical Implications :
2.2.2. Iloperidone
- Iloperidone shares risperidone’s piperidine and fluorophenyl groups but replaces benzisoxazole with a benzimidazole ring .
- Metabolism : Both drugs are metabolized by CYP2D6 and CYP3A4, but iloperidone has a higher risk of QTc prolongation due to additional potassium channel inhibition .
Metabolic and Pharmacokinetic Comparisons
Key metabolic differences among related compounds:
Compound | Primary Metabolizing Enzymes | CYP2D6 Inhibition (Ki, μM) | CYP3A4 Inhibition (Ki, μM) |
---|---|---|---|
Risperidone | CYP2D6, CYP3A4 | 6.9 | 67 |
9-Hydroxyrisperidone | N/A | 16 | 80 |
Ziprasidone | CYP3A4 | 11 | 64 |
Key Findings :
- Risperidone and its metabolite 9-hydroxyrisperidone show stronger CYP2D6 inhibition than ziprasidone, increasing the risk of drug-drug interactions .
- Dose adjustments for risperidone are recommended in CYP2D6 poor metabolizers (26% reduction in Asians vs. 45% in Caucasians) .
Clinical Efficacy Comparisons
- Relapse Prevention :
- Adjunct Therapy :
- In chronic schizophrenia, risperidone addition to neuroleptics improved symptoms more than mosapramine (a D₂-selective agent), likely due to its 5-HT₂A/D₂ dual antagonism .
Q & A
Basic Research Questions
Q. What experimental designs are optimal for synthesizing and characterizing Risperidone E-Oxime with high purity?
- Methodology : Utilize a Box-Behnken design to optimize critical variables (e.g., reaction temperature, solvent ratios, catalyst concentration) and minimize process variability. For structural characterization, employ FTIR spectroscopy to confirm functional groups (e.g., oxime formation) and HPLC (e.g., PerkinElmer LC 300 system) to assess purity and detect impurities .
- Key Considerations : Ensure compatibility studies between this compound and excipients using thermal analysis (DSC/TGA) to avoid interactions that compromise stability .
Q. How can researchers validate the identity and purity of this compound in novel formulations?
- Methodology : Combine mass spectrometry (MS) and nuclear magnetic resonance (NMR) for structural elucidation. For purity, apply United States Pharmacopeia (USP) guidelines using HPLC with photodiode array detection to quantify impurities at thresholds ≤0.1% .
- Data Interpretation : Cross-reference spectral data with published benchmarks (e.g., this compound CAS 691007-09-7) and ensure compliance with ICH Q2(R1) validation guidelines .
Advanced Research Questions
Q. What strategies resolve contradictions in pharmacokinetic data for this compound across preclinical studies?
- Methodology : Conduct meta-analysis with stratified subgrouping (e.g., species, dosing regimens) to identify confounding variables. Use ANOVA (SPSS/GraphPad Prism) to compare dose-dependent effects, as demonstrated in osteoblast studies .
- Data Contradiction Analysis : Apply sensitivity analysis to isolate factors like bioavailability differences due to formulation excipients (e.g., mannitol vs. microcrystalline cellulose) or metabolic enzyme variability .
Q. How can Quality by Design (QbD) principles optimize this compound formulation for pediatric use?
- Methodology : Define critical quality attributes (CQAs) (e.g., disintegration time, dose uniformity) and critical process parameters (CPPs) (e.g., compression force, glidant concentration). Use response surface modeling to predict optimal excipient ratios (e.g., Avicel PH101, Aerosil) for mini-tablet formulations .
- Validation : Employ in vitro-in vivo correlation (IVIVC) to link dissolution profiles (simulated salivary fluid) with bioavailability data, ensuring compliance with pediatric dosing requirements .
Q. What frameworks are effective for formulating hypothesis-driven research questions on this compound’s neuropharmacological mechanisms?
- Methodology : Apply the FINER criteria (Feasible, Interesting, Novel, Ethical, Relevant) and PICO framework (Population, Intervention, Comparison, Outcome). For example:
- Population: In vitro neuronal models.
- Intervention: this compound exposure at IC50 concentrations.
- Comparison: Parent compound (Risperidone).
- Outcome: Dopamine D2 receptor binding affinity .
Q. Methodological Guidance
Q. How should researchers address variability in this compound’s physicochemical properties during formulation development?
- Approach : Perform Design of Experiments (DoE) to evaluate interactions between variables (e.g., particle size, flowability). For example, a three-factor, three-level Box-Behnken design can optimize superdisintegrant concentration and glidant surface area to enhance tablet robustness .
- Statistical Tools : Use multivariate regression (Minitab/JMP) to model relationships between variables and outcomes like friability (<1%) and disintegration time (<60 seconds) .
Q. What ethical and reproducibility standards apply to preclinical studies on this compound?
- Guidelines : Adhere to ARRIVE 2.0 guidelines for experimental reporting. Include raw data in supplementary materials (e.g., REDCap datasets) and document statistical power calculations to justify sample sizes .
- Reproducibility : Provide detailed synthesis protocols (e.g., reaction stoichiometry, purification steps) and instrumental parameters (e.g., HPLC column type, gradient program) to enable independent replication .
Properties
Molecular Formula |
C23H28F2N4O2 |
---|---|
Molecular Weight |
430.5 g/mol |
IUPAC Name |
3-[2-[4-[C-(2,4-difluorophenyl)-N-hydroxycarbonimidoyl]piperidin-1-yl]ethyl]-2-methyl-6,7,8,9-tetrahydropyrido[1,2-a]pyrimidin-4-one |
InChI |
InChI=1S/C23H28F2N4O2/c1-15-18(23(30)29-10-3-2-4-21(29)26-15)9-13-28-11-7-16(8-12-28)22(27-31)19-6-5-17(24)14-20(19)25/h5-6,14,16,31H,2-4,7-13H2,1H3 |
InChI Key |
BRCINVRBDDVLDW-UHFFFAOYSA-N |
Canonical SMILES |
CC1=C(C(=O)N2CCCCC2=N1)CCN3CCC(CC3)C(=NO)C4=C(C=C(C=C4)F)F |
Origin of Product |
United States |
Synthesis routes and methods I
Procedure details
Synthesis routes and methods II
Procedure details
Synthesis routes and methods III
Procedure details
Disclaimer and Information on In-Vitro Research Products
Please be aware that all articles and product information presented on BenchChem are intended solely for informational purposes. The products available for purchase on BenchChem are specifically designed for in-vitro studies, which are conducted outside of living organisms. In-vitro studies, derived from the Latin term "in glass," involve experiments performed in controlled laboratory settings using cells or tissues. It is important to note that these products are not categorized as medicines or drugs, and they have not received approval from the FDA for the prevention, treatment, or cure of any medical condition, ailment, or disease. We must emphasize that any form of bodily introduction of these products into humans or animals is strictly prohibited by law. It is essential to adhere to these guidelines to ensure compliance with legal and ethical standards in research and experimentation.