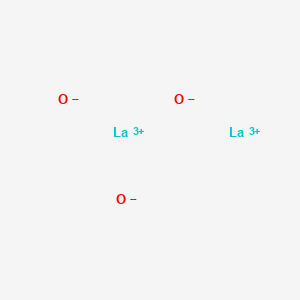
Lanthanum oxide
Overview
Description
Lanthanum oxide (La₂O₃) is a white, odorless solid with a molecular weight of 325.81 g/mol and a density of 6.51 g/cm³ . It is insoluble in water but dissolves in dilute acids, forming lanthanum salts . Its high melting point (2315°C) and thermal stability make it suitable for high-temperature applications, such as optical glass, ceramics, and catalysts .
Synthesis: La₂O₃ is primarily extracted from monazite sand [(REE, Th)PO₄], involving digestion with sulfuric acid, separation of cerium (Ce) and neodymium (Nd) via selective precipitation, and calcination of lanthanum oxalate . Certified reference materials (CRMs) of La₂O₃ achieve purities up to 99.995%, with impurities like Ce(OH)₃ and Nd₂Y₂O₃F₁₆ .
Preparation Methods
Synthetic Routes and Reaction Conditions
Lanthanum oxide can be synthesized through several methods:
-
Spray Pyrolysis: : This method involves spraying a solution of lanthanum chloride onto a preheated substrate, resulting in the formation of lanthanum hydroxide, which is then dehydrated to form lanthanum(III) oxide .
Reaction: [ 2 \text{LaCl}_3 + 3 \text{H}_2\text{O} \rightarrow 2 \text{La(OH)}_3 + 6 \text{HCl} ] [ 2 \text{La(OH)}_3 \rightarrow \text{La}_2\text{O}_3 + 3 \text{H}_2\text{O} ]
-
Sol-Gel Method: : This technique involves the hydrolysis and condensation of lanthanum alkoxides, followed by calcination at high temperatures to obtain lanthanum(III) oxide .
-
Thermal Decomposition: : this compound nanoparticles can be prepared by the thermal decomposition of lanthanum(III) supramolecular compounds at high temperatures .
Industrial Production Methods
In industrial settings, lanthanum(III) oxide is typically produced by the thermal decomposition of lanthanum carbonate or lanthanum nitrate. The process involves heating these compounds to high temperatures to drive off carbon dioxide or nitrogen oxides, leaving behind lanthanum(III) oxide .
Chemical Reactions Analysis
Types of Reactions
Oxidation: Lanthanum oxide can undergo oxidation reactions, although it is already in a high oxidation state.
Reduction: It can be reduced to lanthanum metal using strong reducing agents.
Substitution: this compound can react with various acids to form lanthanum salts.
Common Reagents and Conditions
Acids: this compound reacts with acids such as hydrochloric acid to form lanthanum chloride
Biological Activity
Lanthanum oxide (La₂O₃) is a rare earth metal oxide that has garnered attention for its diverse biological activities, particularly in the fields of medicine and environmental science. This article explores the biological activity of this compound, focusing on its cytotoxic properties, antibacterial effects, and potential applications in agriculture and environmental remediation.
1. Cytotoxicity and Cancer Therapy
Recent studies have highlighted the potential of this compound nanoparticles (La₂O₃ NPs) as therapeutic agents in oncology.
Key Findings:
- Mechanism of Action: La₂O₃ NPs exhibit cytotoxic effects on glioblastoma cells by enhancing the efficacy of radiation therapy and temozolomide (TMZ). The nanoparticles enter cells via clathrin-mediated endocytosis, leading to apoptosis through both intrinsic and extrinsic pathways, as well as inducing DNA damage and autophagy .
- Experimental Evidence: In vitro experiments demonstrated that increasing concentrations of La₂O₃ NPs correlated with higher levels of reactive oxygen species (ROS) and apoptosis markers. These findings suggest that La₂O₃ NPs can sensitize cancer cells to conventional therapies, potentially improving treatment outcomes for aggressive tumors like glioblastoma .
2. Antibacterial Activity
This compound has shown promising antibacterial properties, making it a candidate for use in antimicrobial applications.
Research Insights:
- Synthesis and Structure: Various morphologies of La₂O₃ (e.g., nanospindles, nanopolyhedra, and nanospheres) were synthesized to evaluate their antibacterial effectiveness. Spherical La₂O₃ exhibited superior phosphate removal capabilities and antibacterial activity compared to other shapes .
- Mechanism: The antibacterial action is believed to be linked to the nanoparticles' ability to remove phosphates from microbial growth media, which is essential for bacterial survival .
3. Environmental Impact and Aquatic Toxicity
The ecological safety of this compound nanoparticles has been assessed through toxicity studies on aquatic organisms.
Toxicity Findings:
- Aquatic Species Tested: The acute toxicity of La₂O₃ NPs was evaluated on freshwater microalgae (Chlorella sp.) and crustaceans (Daphnia magna). Results indicated no significant toxic effects on Chlorella sp. at concentrations up to 1000 mg/L, while Daphnia magna exhibited considerable toxicity at higher concentrations (EC50 = 500 mg/L) .
- Implications for Environmental Safety: These findings are crucial for risk assessment strategies regarding the environmental release of this compound nanoparticles .
4. Agricultural Applications
This compound nanoparticles have shown potential benefits in agricultural practices.
Effects on Plant Growth:
- Enhancement of Germination: In vitro studies reported that La₂O₃-NPs significantly improved seed germination rates and plant growth parameters in Citrus limon seeds. The nanoparticles promoted root and shoot development, supporting photosynthesis and stress resistance in plants .
- Mechanisms Involved: The positive effects on plant growth may be attributed to enhanced nutrient uptake facilitated by the unique properties of La₂O₃-NPs .
5. Summary Table of Biological Activities
Scientific Research Applications
Optical Materials
Enhancement of Optical Glass:
Lanthanum oxide is widely used in the production of optical glass to improve its alkali resistance. It enhances several properties, including:
- Density: Increases the overall density of glass.
- Refractive Index: Improves light transmission and reduces optical distortion.
- Hardness: Contributes to the durability of optical components.
These enhancements make this compound a critical component in high-performance lenses and other optical devices .
Ceramics
Modification of Ceramic Properties:
this compound is used to modify the properties of ceramics, particularly those produced with galvanic sludge additives. Its incorporation can lead to:
- Increased chemical resistance.
- Improved mechanical strength.
- Enhanced thermal stability.
A study demonstrated that ceramics containing this compound exhibited superior performance in harsh environments compared to traditional materials .
Catalysis
FCC Catalysts:
In the field of catalysis, this compound is utilized in Fluid Catalytic Cracking (FCC) processes. It serves as a catalyst support that enhances the activity and selectivity of catalytic reactions, particularly in petroleum refining .
Nanotechnology
Nanoparticles Applications:
this compound nanoparticles are emerging as valuable materials in various fields due to their high photoelectric conversion efficiency. Key applications include:
- Biological Analysis: Utilized in DNA and protein analysis due to their biocompatibility.
- Electronics: Potential applications in future CMOS (Complementary Metal-Oxide-Semiconductor) technologies and non-volatile memory systems (NVMS) due to their electrical properties .
Electronics
Dielectric Materials:
this compound is employed as a dielectric material in capacitors and other electronic components. Its high dielectric constant makes it suitable for miniaturized electronic devices, contributing to improved performance and efficiency .
Health and Environmental Applications
Potential Health Implications:
Research indicates that this compound may have implications in pulmonary disease studies, particularly regarding its nanoparticle form's interaction with biological systems. However, further studies are needed to fully understand these effects .
Summary Table of Applications
Application Area | Specific Uses | Key Benefits |
---|---|---|
Optical Materials | Alkali-resistant glass | Improved density, refractive index |
Ceramics | Chemically resistant ceramics | Enhanced strength, thermal stability |
Catalysis | FCC processes | Increased reaction efficiency |
Nanotechnology | DNA/protein analysis, electronics | High photoelectric efficiency |
Electronics | Dielectric materials | High dielectric constant |
Health & Environment | Pulmonary disease research | Potential insights into health effects |
Case Studies
-
Optical Glass Enhancement:
A study by Stanford Advanced Materials highlighted the effectiveness of this compound in improving the alkali resistance of optical glass used in high-end cameras and telescopes, resulting in clearer images and longer-lasting equipment . -
Ceramic Modification:
Research conducted on ceramics incorporating galvanic sludge demonstrated that adding this compound significantly improved their chemical resistance, making them suitable for use in aggressive environments such as chemical processing plants . -
Nanoparticle Applications:
Investigations into this compound nanoparticles revealed their potential for use in advanced electronic devices, with ongoing research focusing on optimizing their synthesis for enhanced performance in future technologies .
Q & A
Basic Research Questions
Q. What are the established methods for synthesizing high-purity lanthanum oxide from monazite sand?
this compound is synthesized through multi-stage processes involving monazite sand digestion, rare-earth hydroxide precipitation, selective separation (e.g., Ce and Nd), oxalate precipitation, and calcination. Key steps include:
- Digestion : Monazite sand is fused with NaOH or H₂SO₄ to dissolve phosphate bonds .
- Separation : Ce is oxidized to Ce⁴⁺ and separated via pH-controlled precipitation, while Nd is removed using solvent extraction .
- Calcination : Lanthanum oxalate is heated at 800–1000°C to yield La₂O₃ . Methodological challenges include minimizing contamination from Th and U during digestion and ensuring stoichiometric control during calcination .
Q. How do ionic radii and coordination numbers influence the structural properties of La₂O₃?
Shannon’s revised ionic radii data (1976) indicates La³⁺ has an effective ionic radius of 1.032 Å in 6-coordination, expanding to 1.160 Å in 9-coordination. This variability affects lattice stability, dielectric properties, and reactivity in applications like catalysis or optical materials. Polyhedral distortion and covalence further shorten bond lengths (e.g., La³⁺-O bonds in distorted octahedra) .
Q. What analytical techniques are used to characterize La₂O₃ purity and crystallinity?
- X-ray diffraction (XRD) : Identifies phases like La₂O₃, Ce(OH)₃, or Nd₂Y₂O₃F₁₆ impurities .
- X-ray fluorescence (XRF) : Quantifies major oxides (La₂O₃, Nd₂O₃) and trace elements (Sm, Y) .
- Thermogravimetric analysis (TGA) : Measures water content (<1% for certified reference materials) .
Advanced Research Questions
Q. How can researchers reconcile discrepancies in La₂O₃ purity data across laboratories?
Interlaboratory certification using ISO 35-2006 statistical methods resolves inconsistencies. For example, La₂O₃ concentrations from seven labs ranged from 91.190% to 95.112%, but ISO averaging yielded 91.662 ± 0.007% . Contradictions arise from:
- Methodological variability : XRF calibration differences.
- Impurity retention : Residual Ce(OH)₃ or Nd₂Y₂O₃F₁₆ from incomplete separation . Standardized protocols for digestion and homogeneity testing (e.g., 10 subsamples analyzed in duplicate) reduce uncertainty .
Q. What experimental designs optimize La₂O₃’s role in solid oxide fuel cell (SOFC) cathodes?
La₂O₃-doped perovskites (e.g., LaSrMnO₃) enhance oxygen reduction kinetics. Key factors include:
- Microstructure control : Grain boundary engineering via ball milling or thin-film deposition.
- Ionic conductivity : Measured via electrochemical impedance spectroscopy (EIS) at 600–800°C . Advanced studies use density functional theory (DFT) to predict dopant effects on oxygen vacancy formation .
Q. How does La₂O₃’s optical bandgap (4.3 eV) impact its use in waveguides or luminescent materials?
The wide bandgap enables UV transparency and high refractive index (~2.0). For luminescence:
- Doping : Bi³⁺-activated La₂O₃ emits blue-violet light, with excitation spectra sensitive to crystal field distortion .
- Fabrication : Sol-gel methods produce nanocrystalline La₂O₃ with tunable bandgaps via annealing .
Q. What statistical methods ensure homogeneity and stability in La₂O₃ reference materials?
- Homogeneity : Subsamples analyzed via XRF (n=10) must show standard deviation (Ss) ≤ 0.3σ, where σ is derived from Horwitz’s equation .
- Stability : Accelerated aging tests (40°C, 75% humidity) confirm |Xₜ − Yₜ| ≤ 0.3σ over 6 months .
Q. Why do La₂O₃ epitaxial thin films exhibit superconductivity at 5 K, and how is this engineered?
Rock-salt structure LaO thin films (grown via molecular beam epitaxy) show metallic conductivity and superconductivity due to electron-phonon coupling. Key parameters:
- Substrate choice : Lattice-matched substrates (e.g., MgO) minimize strain.
- Defect engineering : Oxygen vacancies enhance carrier density .
Q. Methodological Considerations
Q. How are water content and hygroscopicity controlled during La₂O₃ storage?
- Drying : Samples heated at 110°C for 1 hour achieve <1% water content .
- Storage : Desiccators with silica gel prevent rehydration, critical for catalytic or electronic applications .
Q. What protocols validate La₂O₃’s catalytic efficiency in methane reforming or CO₂ reduction?
- Activity tests : Fixed-bed reactors with gas chromatography (GC) quantify CH₄ conversion or CO selectivity.
- Surface analysis : X-ray photoelectron spectroscopy (XPS) identifies active La³⁺-O sites .
Comparison with Similar Compounds
Ionic Radii and Structural Effects
Lanthanum’s ionic radius (La³⁺: 1.16 Å for coordination number 6) is larger than Ce³⁺ (1.14 Å) and Nd³⁺ (1.12 Å), influencing crystal lattice stability and bond lengths . Ce⁴⁺ (0.97 Å) in cerium oxide (CeO₂) enables redox activity, while La³⁺’s size stabilizes high-coordination structures like hexagonal La₂O₃ .
Thermal and Physical Properties
Property | La₂O₃ | CeO₂ | Nd₂O₃ | La₂TiO₇ |
---|---|---|---|---|
Melting Point | 2315°C | ~2400°C | ~2233°C | >1800°C |
Density | 6.51 g/cm³ | 7.65 g/cm³ | 7.24 g/cm³ | 5.9–6.2 g/cm³ |
Ionic Radius | 1.16 Å (La³⁺) | 0.97 Å (Ce⁴⁺) | 1.12 Å (Nd³⁺) | – |
Key Differences :
- CeO₂ : Higher density and redox versatility (Ce⁴⁺ ↔ Ce³⁺) make it superior for oxygen storage and catalytic oxidation .
- Nd₂O₃ : Lower thermal stability but critical for Nd-Fe-B magnets and laser glass .
- La₂TiO₇ : A perovskite-structured mixed oxide with applications in dielectric materials, though less thermally stable than La₂O₃ .
Reactivity and Catalytic Performance
La₂O₃ exhibits facet-dependent reactivity. The (110) facet has the lowest methane dissociation barrier (0.68 eV) and strongest oxygen adsorption (-0.31 eV), promoting OCM activity . In contrast, CeO₂’s oxygen mobility enhances CO oxidation and automotive catalytic converters, while La₂O₃’s basicity favors hydrocarbon activation .
Properties
IUPAC Name |
lanthanum(3+);oxygen(2-) | |
---|---|---|
Source | PubChem | |
URL | https://pubchem.ncbi.nlm.nih.gov | |
Description | Data deposited in or computed by PubChem | |
InChI |
InChI=1S/2La.3O/q2*+3;3*-2 | |
Source | PubChem | |
URL | https://pubchem.ncbi.nlm.nih.gov | |
Description | Data deposited in or computed by PubChem | |
InChI Key |
MRELNEQAGSRDBK-UHFFFAOYSA-N | |
Source | PubChem | |
URL | https://pubchem.ncbi.nlm.nih.gov | |
Description | Data deposited in or computed by PubChem | |
Canonical SMILES |
[O-2].[O-2].[O-2].[La+3].[La+3] | |
Source | PubChem | |
URL | https://pubchem.ncbi.nlm.nih.gov | |
Description | Data deposited in or computed by PubChem | |
Molecular Formula |
La2O3 | |
Record name | lanthanum(III) oxide | |
Source | Wikipedia | |
URL | https://en.wikipedia.org/wiki/Lanthanum(III)_oxide | |
Description | Chemical information link to Wikipedia. | |
Source | PubChem | |
URL | https://pubchem.ncbi.nlm.nih.gov | |
Description | Data deposited in or computed by PubChem | |
Molecular Weight |
325.809 g/mol | |
Source | PubChem | |
URL | https://pubchem.ncbi.nlm.nih.gov | |
Description | Data deposited in or computed by PubChem | |
Physical Description |
Dry Powder; Other Solid; Pellets or Large Crystals, White solid; Insoluble in water; [Merck Index] Soluble in acids; [Hawley] | |
Record name | Lanthanum oxide (La2O3) | |
Source | EPA Chemicals under the TSCA | |
URL | https://www.epa.gov/chemicals-under-tsca | |
Description | EPA Chemicals under the Toxic Substances Control Act (TSCA) collection contains information on chemicals and their regulations under TSCA, including non-confidential content from the TSCA Chemical Substance Inventory and Chemical Data Reporting. | |
Record name | Lanthanum oxide | |
Source | Haz-Map, Information on Hazardous Chemicals and Occupational Diseases | |
URL | https://haz-map.com/Agents/2131 | |
Description | Haz-Map® is an occupational health database designed for health and safety professionals and for consumers seeking information about the adverse effects of workplace exposures to chemical and biological agents. | |
Explanation | Copyright (c) 2022 Haz-Map(R). All rights reserved. Unless otherwise indicated, all materials from Haz-Map are copyrighted by Haz-Map(R). No part of these materials, either text or image may be used for any purpose other than for personal use. Therefore, reproduction, modification, storage in a retrieval system or retransmission, in any form or by any means, electronic, mechanical or otherwise, for reasons other than personal use, is strictly prohibited without prior written permission. | |
CAS No. |
1312-81-8 | |
Record name | Lanthanum oxide | |
Source | ChemIDplus | |
URL | https://pubchem.ncbi.nlm.nih.gov/substance/?source=chemidplus&sourceid=0001312818 | |
Description | ChemIDplus is a free, web search system that provides access to the structure and nomenclature authority files used for the identification of chemical substances cited in National Library of Medicine (NLM) databases, including the TOXNET system. | |
Record name | Lanthanum oxide (La2O3) | |
Source | EPA Chemicals under the TSCA | |
URL | https://www.epa.gov/chemicals-under-tsca | |
Description | EPA Chemicals under the Toxic Substances Control Act (TSCA) collection contains information on chemicals and their regulations under TSCA, including non-confidential content from the TSCA Chemical Substance Inventory and Chemical Data Reporting. | |
Record name | Lanthanum oxide | |
Source | European Chemicals Agency (ECHA) | |
URL | https://echa.europa.eu/substance-information/-/substanceinfo/100.013.819 | |
Description | The European Chemicals Agency (ECHA) is an agency of the European Union which is the driving force among regulatory authorities in implementing the EU's groundbreaking chemicals legislation for the benefit of human health and the environment as well as for innovation and competitiveness. | |
Explanation | Use of the information, documents and data from the ECHA website is subject to the terms and conditions of this Legal Notice, and subject to other binding limitations provided for under applicable law, the information, documents and data made available on the ECHA website may be reproduced, distributed and/or used, totally or in part, for non-commercial purposes provided that ECHA is acknowledged as the source: "Source: European Chemicals Agency, http://echa.europa.eu/". Such acknowledgement must be included in each copy of the material. ECHA permits and encourages organisations and individuals to create links to the ECHA website under the following cumulative conditions: Links can only be made to webpages that provide a link to the Legal Notice page. | |
Record name | Lanthanum oxide | |
Source | European Chemicals Agency (ECHA) | |
URL | https://echa.europa.eu/information-on-chemicals | |
Description | The European Chemicals Agency (ECHA) is an agency of the European Union which is the driving force among regulatory authorities in implementing the EU's groundbreaking chemicals legislation for the benefit of human health and the environment as well as for innovation and competitiveness. | |
Explanation | Use of the information, documents and data from the ECHA website is subject to the terms and conditions of this Legal Notice, and subject to other binding limitations provided for under applicable law, the information, documents and data made available on the ECHA website may be reproduced, distributed and/or used, totally or in part, for non-commercial purposes provided that ECHA is acknowledged as the source: "Source: European Chemicals Agency, http://echa.europa.eu/". Such acknowledgement must be included in each copy of the material. ECHA permits and encourages organisations and individuals to create links to the ECHA website under the following cumulative conditions: Links can only be made to webpages that provide a link to the Legal Notice page. | |
Record name | LANTHANUM OXIDE | |
Source | FDA Global Substance Registration System (GSRS) | |
URL | https://gsrs.ncats.nih.gov/ginas/app/beta/substances/4QI5EL790W | |
Description | The FDA Global Substance Registration System (GSRS) enables the efficient and accurate exchange of information on what substances are in regulated products. Instead of relying on names, which vary across regulatory domains, countries, and regions, the GSRS knowledge base makes it possible for substances to be defined by standardized, scientific descriptions. | |
Explanation | Unless otherwise noted, the contents of the FDA website (www.fda.gov), both text and graphics, are not copyrighted. They are in the public domain and may be republished, reprinted and otherwise used freely by anyone without the need to obtain permission from FDA. Credit to the U.S. Food and Drug Administration as the source is appreciated but not required. | |
Synthesis routes and methods I
Procedure details
Synthesis routes and methods II
Procedure details
Synthesis routes and methods III
Procedure details
Synthesis routes and methods IV
Procedure details
Disclaimer and Information on In-Vitro Research Products
Please be aware that all articles and product information presented on BenchChem are intended solely for informational purposes. The products available for purchase on BenchChem are specifically designed for in-vitro studies, which are conducted outside of living organisms. In-vitro studies, derived from the Latin term "in glass," involve experiments performed in controlled laboratory settings using cells or tissues. It is important to note that these products are not categorized as medicines or drugs, and they have not received approval from the FDA for the prevention, treatment, or cure of any medical condition, ailment, or disease. We must emphasize that any form of bodily introduction of these products into humans or animals is strictly prohibited by law. It is essential to adhere to these guidelines to ensure compliance with legal and ethical standards in research and experimentation.